There are three main mechanisms for anemia:
- Hemorrhage: This is due to loss of RBCs outside vessels. These anemias are usually regenerative (given sufficient time for a bone marrow response). Hemorrhage can be:
- Internal: Into a tissue or body cavity, such as the peritoneum.
- External: In this case, blood (RBC, plasma) is lost from the body, e.g. hemorrhage from the skin or into gastrointestinal, urinary, respiratory tracts.
- Hemolysis: This is due to destruction of RBCs within the body before they have finished their normal lifespan. These anemias are usually regenerative (given sufficient time for a bone marrow response) but may be non-regenerative or inadequately regenerative if the disease concurrently suppresses bone marrow erythropoiesis. The RBCs are usually removed by macrophages in a process called extravascular hemolysis, typically in the spleen, liver and bone marrow. The macrophages phagocytize the RBC prematurely because the RBCs are abnormal (e.g. have antibody attached to them, are more rigid, etc) or macrophages are doing something they are not supposed to because they are excessively activated (e.g. by inflammatory cytokines, such as interferon-γ) or abnormal (e.g. erythrophagocytic histiocytic sarcoma, which is a tumor of macrophages, where macrophages phagocytize excess numbers of normal RBCs).
In some patients with hemolytic anemia (depending on the cause and the patient), there can be a concurrent intravascular hemolysis. This is when RBCs lyse (“pop”) in the circulation, releasing hemoglobin into plasma (hemoglobinemia) that then spills into urine (resulting in hemoglobinuria). Thus, intravascular hemolysis is identified by hemoglobinemia (free hemoglobin in serum or plasma) and hemoglobinuria (free hemoglobin in urine) in a given patient with anemia. These two forms of hemolysis are outlined more in detail below. - Decreased bone marrow production: These anemias are usually non-regenerative.
Decreased production can be due to:- Intramedullary disease: A problem within the bone marrow preventing a bone marrow response, e.g. acute leukemia.
- Extramedullary disease: A disease outside the bone marrow that secondarily suppresses the ability of the bone marrow to respond to an anemia or produce erythrocytes.
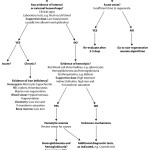
Assessment of regeneration is the first step in working up an anemia and helps identify the mechanism for an anemia. A regenerative anemia typically results from loss of RBC (hemorrhage) or premature destruction of RBC (hemolysis). These mechanisms can be differentiated on the basis of history, clinical findings, and other laboratory results (RBC morphologic features, total protein, iron and percentage iron saturation, bilirubin concentration) – see diagnostic algorithm. An unexpectedly low or inadequate regenerative response in an anemia that is primarily due to hemorrhage or hemolysis should prompt a search for underlying causes of bone marrow suppression, e.g. inflammatory disease (as long as the bone marrow has had time to respond to the anemia).
Note:
- A regenerative response may be seen in anemia of inflammatory disease, which is mostly due to cytokine-suppression of erythropoiesis in the bone marrow (cytokines such as interleukin-1, tumor necrosis factor-α, and IFNγ) and iron sequestration, as well as a hemolytic process. The regeneration may be due to so-called “stress” erythropoiesis, which occurs in the spleen (in mice at any rate) and compensates for the suppression of bone marrow erythropoiesis. Stress erythropoiesis can be episodic. (Paulson et al 2020). For more on stress erythropoiesis, refer to the erythropoiesis page.
- We cannot always determine the mechanism of an anemia in a given animal. In an animal with a regenerative anemia and no clear evidence for hemorrhage or hemolysis, consider stress erythropoiesis, inflammation-induced hemolysis, or stimulation of erythropoiesis from systemic or renal hypoxia.
Hemorrhage
RBCs can be lost externally through the gastrointestinal, respiratory or urinary tracts or skin or “lost” internally into a body cavity (e.g. hemoperitoneum). External and internal blood loss can be acute or chronic. With acute hemorrhage, the total protein and hematocrit or packed cell volume may be normal due to equal losses of both protein and red blood cells. If the hemorrhage is severe, the animal should show clinical signs of hypoxia (weakness) and anemia (pallor), however. Within 4-12 hours, the total protein concentration should be decreased due to compensatory mechanisms to restore blood volume (fluid shifts from the extravascular to the intravascular space, ADH secretion, aldosterone secretion to stimulate sodium and water resorption in the kidneys). The HCT/PCV may be normal due to splenic contraction, particularly in animals with muscular spleens. By 24 hours, both total protein concentration and HCT/PCV should be decreased. Typical laboratory findings with hemorrhage are a low total protein concentration, which is often due to concurrent decreases in albumin and globulins, particularly with external hemorrhage (because the animal is losing the protein along with red blood cells and iron). However, albumin is more reliably decreased in studies of animals with induced hemorrhage via blood withdrawal (Elman 1939, Elman et al 1944). Retrospective studies of spontaneous hemoperitoneum in dogs and cats (e.g. from trauma or ruptured splenic hemangiosarcoma) have shown low protein concentrations in 42% and 36% of dogs (Lux et al 2013) and cats (Kulp et al 2010), respectively, with hypoalbuminemia in more animals (78% of dogs and 55% of cats in the same studies). In one study of 19 horses with acute hemoabdomen, low total protein and low albumin concentrations were seen in 88% and 53% respectively (globulins were not mentioned) (Pusterla et al 2006). In another study of 67 horses with acute hemoperitoneum, the median total protein concentration was 5.5 g/dL (range, 3.3-8.7 g/dL) with a median HCT of 31% (mild anemia with our reference interval, range, 11-73%) (Dechant et al 2006). Thus, protein, albumin and globulin concentrations may not always be low with hemorrhage and their presence depends on multiple factors, including the degree and duration of hemorrhage, need to maintain intravascular volume, the ability of the body to increase protein production in states of chronic or ongoing hemorrhage, and concurrent inflammation (which may depress albumin concentrations further, while increasing globulin concentrations).
With chronic external hemorrhage, in which the animal is losing iron along with RBCs, iron deficiency may compound the anemia, but only once iron stores in the body are depleted (at this stage, a low iron concentration and % transferrin saturation should be seen on a chemistry panel). Iron deficiency decreases red blood cell production and will dampen any regenerative response to the hemorrhage. Iron deficiency may manifest as a regenerative or non-regenerative anemia. With iron deficiency, smaller RBCs containing less hemoglobin are produced, thus an iron deficiency anemia is usually (not always) microcytic and hypochromic and hypochromasia is evident on a blood smear. In fact, hypochromasia or hypochromic RBCs usually precedes a microcytosis in iron deficiency anemias. With iron deficiency anemias, there may also be evidence of RBC fragmentation, such acanthocytes, schistocytes and keratocytes, in blood smears (particularly in dogs, pigs and woodchucks) – the fragmentation is attributed to mechanical fragility of the RBCs.
Note:
- Iron deficiency does not result from acute hemorrhage (iron stores are not depleted) or internal hemorrhage (iron is not “lost” from the body but recycled internally).
- With internal hemorrhage into the peritoneal space, RBCs are actually re-absorbed mostly intact (90% within 4 days), thus it mimics an auto-transfusion (Allcock 1962).
Hemolysis
Hemolysis indicates reduced RBC lifespan due to destruction of RBC. Red blood cells are destroyed when they are prematurely removed from the circulation by macrophages, which phagocytize the cells before their normal lifespan is up. This is called extravascular hemolysis and the phagocytosis by macrophages is occurring within the spleen, in particular, but also other organs such as the liver and bone marrow. Extravascular hemolysis (phagocytosis of RBC by macrophages) is always occurring in a hemolytic anemia. In a few animals with hemolytic anemia, their RBC may also rupture (“pop”) within blood vessels. This is called intravascular hemolysis but it does not occur in all cases of hemolytic anemia, only in some unfortunate patients with specific causes of hemolytic anemia (e.g. oxidant injury, immune-mediated hemolytic anemia). Thus, all patients with hemolytic anemia have extravascular hemolysis (usually the major reason why they are anemic), whereas some patients will have concurrent intravascular hemolysis.
Hemolytic anemia (whether there is an intravascular component or not) can result in icterus, i.e. increased total bilirubin concentration, which is mostly indirect (unconjugated) bilirubin (the porphyrin ring of hemoglobin is converted into unconjugated bilirubin within macrophages). The increase in total and indirect bilirubin concentration will only occur if macrophage production of unconjugated bilirubin exceeds the ability of the liver to take it up, thus increased indirect and total bilirubin concentrations are not invariably present in an animal with a hemolytic anemia. We can also see high iron concentrations and percentage transferrin or iron saturation (total iron binding capacity is normal) in a hemolytic anemia. This is because iron is high in RBCs and iron concentrations increase in serum/plasma with increased RBC turnover (as occurs in a hemolytic anemia), The total protein concentration is usually not decreased like it can be in hemorrhage (although albumin concentrations may be decreased due to an inflammatory response). Also, a hemolytic anemia can induce a stronger regenerative response than a hemorrhagic anemia (particularly that due to external hemorrhage) and if a blood smear is examined, a cause for the hemolytic anemia may be identified (e.g. RBC shape change, presence of an erythroparasite).
Extravascular hemolysis
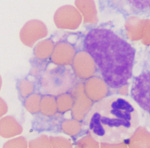
Extravascular hemolysis occurs when RBCs are phagocytized by macrophages in the spleen, liver and bone marrow (see image of an erythrophage to the right). Extravascular hemolysis is always present in an animal with a hemolytic anemia. In certain patients with specific diseases, there may be an additional component of intravascular hemolysis (luckily this does not happen too often as intravascular hemolysis is bad for a patient because it can cause acute kidney injury). Note that during the normal aging of red blood cells in the circulation, effete RBCs are destroyed by macrophages, i.e. extravascular hemolysis is always occurring to some degree in our body when RBCs have finished living. This is mediated by phosphatidylserine expression on red blood cells as a consequence of natural cell death (apoptosis or eryptosis) or due to binding of natural antibodies against the red blood cell band 3 protein, which clusters with age (probably due to accumulating oxidative injury). However, this is a physiologic process and does not result in anemia or excessive unconjugated bilirubin production.
With extravascular hemolysis, the erythrocytes are degraded within macrophages (see image above), so hemoglobin is not released free into the cytoplasm. Thus, we do not see hemoglobinemia or hemoglobinuria with extravascular hemolysis alone, unless it is accompanied by intravascular hemolysis.
Causes of extravascular hemolysis
There are many causes of extravascular hemolysis, some of which can be identified by red blood cell changes or other identifiable abnormalities in blood smears.
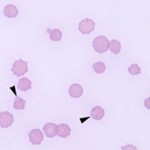
- Immune-mediated hemolytic anemia: This is a common cause of extravascular hemolysis in the dog. Attachment of IgG or IgM causes fixation of complement (to C3b) on red cell membranes. Macrophages possess receptors for the Fc portion of IgG and IgM as well as for C3b, thus causing red blood cells with attached immunoglobulin or C3b to be phagocytized. Partial phagocytosis of erythrocytes forms spherocytes which, in large numbers, are pathognomonic for IMHA. Note, that spherocytes are most readily seen in the dog, because central pallor is usually present in canine erythrocytes. They are difficult to observe in other species. The immunoglobulin- and complement that are attached to or coating the red blood cells can be detected in a direct Coombs test using a Coombs reagent, which consists of species-specific anti-Ig and/or anti-C3. Thus, a positive Coombs test is further supportive evidence of IMHA, but false positives and negatives do occur. IMHA can be primary or secondary to drugs (e.g. penicillin in horses) or erythroparasites.
- Erythroparasites: Many erythroparasites cause a hemolytic anemia due to extravascular hemolysis, e.g. Mycoplasma haemofelis (feline infectious anemia), Anaplasma bovis, Babesia species. With many of these organisms, there is a concurrent immune-mediated component to the anemia (the organisms make the RBC antigenic). Babesia species also induces a concurrent intravascular hemolysis. Note, that not all erythroparasites are associated with an anemia. Candidatum Mycoplasma haemolamae and Mycoplasma haemominutum and turicensis are not always associated with a hemolytic anemia, although the lifespan of RBC with attached organisms is likely reduced.
- Other infectious organisms: Bacteria, such as Leptospira, can cause an extravascular hemolytic anemia, as can rickettsial and viral (e.g. equine infectious anemia) agents. Infections caused by gram negative or positive bacteria (e.g. Staphylococcus aureus, Escherichia coli) do not usually result in extravascular hemolysis unless there is concurrent DIC.
- Oxidant injury: Oxidant injury (e.g. acetaminophen toxicity in cats) can result in extravascular hemolysis. Heinz bodies, eccentrocytes and pyknocytes are seen with oxidant injury (although this is species dependent). The Heinz body-containing red blood cells are removed prematurely from the circulation by macrophages (principally in the spleen). Inherited defects in red cell enzymes that help the red blood cell combat oxidant injury (e.g. glucose-6-phosphate dehydrogenase deficiency in horses) can result in an oxidant-induced hemolytic anemia. As noted above, some forms of oxidant injury (e.g. copper poisoning in sheep, red maple leaf toxicity in horses) can induce concurrent intravascular hemolysis. With intravascular hemolysis, ghost RBCs may be seen in smears (but must be distinguished from artifactual ghosts formed during smear preparation or a consequence of in vitro hemolysis – see more below).
- Fragmentation injury: This usually occurs secondary to vascular disease (e.g. hemangiosarcoma), liver disease or disseminated intravascular coagulation (DIC). Keratocytes, schistocytes, and acanthocytes are observed in peripheral blood in fragmentation anemias. A few spherocytes may be observed in fragmentation anemias and do not indicate immune-mediated disease in this setting. Fragmentation anemias may be non-regenerative as cytokines associated with the primary disease often suppress the bone marrow. Note that some degree of intravascular hemolysis does also occur with fragmentation injury (particularly when fibrin strands shear RBC in DIC), however the amount of hemoglobin released into the circulation is insufficient to cause visible hemoglobinemia or hemoglobinuria. The term “microangiopathic” hemolytic anemia can be used to describe an anemia associated with RBC fragmentation, which is due to small vessel disease, including fibrin thrombi formation (DIC and other causes of microvascular thrombosis), vasculitis and hemangiosarcoma.
- Inflammatory disease: Inflammatory cytokines (such as tumor necrosis factor-α and interferon-γ), complement activation, and oxidant injury from free radicles can decrease RBC lifespan by damaging RBCs and inducing binding of antibodies to RBC surfaces, i.e. the damaged or complement/antibody bound RBCs are prematurely phagocytized by macrophages in the spleen and liver (i.e. extravascular hemolysis). Cytokine-induced activation of macrophages can promote RBC destruction in these sites (Theurl et al 2016, Libregts et al 2011). The cytokines concurrently suppress the bone marrow response and iron is limited, so the anemia may be non-regenerative or inadequately regenerative (unless “stress” erythropoiesis kicks in).
- Histiocytic disorders: In these disorders, instead of RBC destruction occuring due to the RBC being abnormal, they are destroyed because the macrophages are stimulated by cytokines (usually liberated from T cells, i.e. the macrophages are reactive) or are neoplastic (e.g. histiocytic sarcoma). Erythrophagocytic macrophage variants of histiocytic sarcoma (Moore et al 2006) have been identified in dogs (particularly large breeds, like Golden Retrievers and Labradors) and can produce an extravascular hemolytic anemia, that can mimic IMHA.
- Inherited red blood cell defects: Inherited defects of RBC enzymes (e.g. pyruvate kinase deficiency in Beagles and Basenjis) and membranes (e.g. hereditary stomatocytosis) can result in extravascular hemolytic anemias. These have primarily been identified in dogs, but can also occur in other species, including cats (pyruvate kinase deficiency in Abyssinian, Somali and domestic shorthair cats) and cattle (hereditary spherocytosis).
Intravascular hemolysis
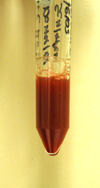
Intravascular hemolysis results from the rupture or lysis of RBC within the circulation, i.e. the RBC are lysing in vivo. When the membrane of erythrocytes rupture, they release their hemoglobin into the plasma. Because hemoglobin concentrations >20 mg/dL will cause visible discoloration of plasma (light pink to dark red, depending on how much hemoglobin is present), hemoglobinemia is usually visible with intravascular hemolysis. When hemoglobin-binding proteins, such as haptoglobin, are saturated, the excess hemoglobin (“free”) spills into urine (one of its fates) so we see concurrent hemoglobinuria. We may also see ghost RBCs in blood smears – these are RBCs that consist only of membrane remnants (ghosts of their former cells) because they have ruptured and released their hemoglobin. However, these are only usually obvious when there is a decent amount of intravascular hemolysis occurring (and ghost RBCs can be an artifact of blood collection or smear preparation). We also see hemoglobinemia in all samples collected from the patient (EDTA, clot tube, heparin, citrate – depending on the tests requested). On our chemistry panels, the hemolytic index is often quite high in patients with intravascular hemolysis (i.e. > 200 units). The image above shows severe hemolysis (red discolored supernatant plasma of blood centrifuged in a microhematocrit tube from EDTA plasma as part of a hemogram, where we assess plasma appearance) in a dog with an immune-mediated hemolytic anemia (the dog has extravascular and intravascular hemolysis). The hemolytic index in such a patient would be > 500 units. The dog concurrently had marked hemoglobinuria as shown in the image to the right. Note that such hemolysis will interfere with clinical pathologic test results, including hemogram results. In an animal with intravascular hemolysis, the result that reflects oxygen-carrying capacity (or oxygen that can be delivered to tissues) on a hemogram is the RBC count because the hemoglobin measurement reflects both that within the RBCs (the machine lyses the intact RBCs to liberate hemoglobin) and that already in plasma (which cannot carry oxygen to tissues). The hematocrit (HCT) may also be accurate as long as the mean cell volume (MCV) is accurate (because the hematocrit is equal to the MCV x RBC count). A packed cell volume (PCV) is likely more accurate than the HCT because it is a directly measured value (as long as we can still manually detect the top of the RBC layer in the microhematocrit tube, which can be difficult in animals with severe hemolysis).
Once hemoglobin is liberated into plasma, the free hemoglobin (which is a tetramer) breaks down into hemoglobin dimers in plasma and has two fates:
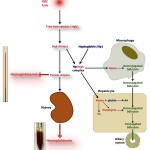
- It can bind haptoglobin (an α-2 globulin produced in the liver) and the hemoglobin-haptoglobin complexes are taken up by hepatocytes and macrophages (the complex binds to the haptoglobin receptor, CD163, initiating phagocytosis), to a lesser extent. Once within macrophages or hepatocytes, the hemoglobin is broken down into unconjugated bilirubin (see image to the right on the sequence of events of how hemoglobin is converted to unconjugated bilirubin). This process is similar to that which occurs when hemoglobin is released from red blood cells that are being destroyed within macrophages during extravascular hemolysis.
- When hemoglobin is in excess of haptoglobin (this occurs at around a hemoglobin concentration of 150 mg/dL), the excess hemoglobin dimers are filtered readily through the glomerulus (free hemoglobin is fairly small, since a hemoglobin monomer is around 17 kD, well below the glomerular filtration barrier limit). This will cause a hemoglobinuria (see image to the right and above) and a positive reaction for heme on the dipstick (with no erythrocytes evident in the urine sediment and no evidence of severe skeletal muscle injury producing myoglobinuria). Since proximal renal tubules can reabsorb hemoglobin from the tubular lumen and contain heme oxygenase, they can generate unconjugated bilirubin.
Thus we can detect the presence of a concurrent intravascular hemolysis if we see hemoglobinemia and hemoglobinuria in an anemic patient. However, we can only make this conclusion, if we rule out in vitro (false) hemolysis first. Intravascular hemolysis is not good for the patient. It results in tissue injury (through initiating oxidant injury and scavenging the important protective vasodilator and platelet inhibitor, nitric oxide), inciting inflammation and triggering thrombosis (heme and hemoglobin bind to toll-like receptor 4 on cells, inciting a similar inflammatory response to lipopolysaccharide from gram negative bacteria). In the kidney, heme/hemoglobin can result in acute kidney injury, which is multifactorial and due to ischemic injury from nitric oxide scavenging and the direct toxic effects of heme. You can actually see the hemoglobin within the tubules in patients with severe intravascular hemolysis and it is called a hemoglobinuric nephropathy. Endothelial dysfunction from heme can result in vasoconstriction and decreased blood flow to organs (including the kidney), compounding tissue injury and immunothrombosis (Sundd et al 2019). Therefore, the presence of intravascular hemolysis in an animal with a hemolytic anemia usually indicates a poorer prognosis.
In vivo versus in vitro hemolysis
Note that RBCs can also lyse or rupture in vitro (either in the blood collection tube or during collection). When this occurs, the hemolysis is considered an artifact and does not indicate the animal has a hemolytic anemia. Artifactual hemolysis results from poor venipuncture technique, prolonged blood storage, exposure to temperature extremes (hot or cold enough to freeze the cells), and certain anticoagulants (fluoride-oxalate) will cause artifactual red blood cell lysis. Red blood cells are also more fragile in lipemic samples and tend to lyse more readily in these samples, even if the blood is stored or handled correctly. This artifactual red blood cell lysis can mimic intravascular hemolysis and it can be very difficult to tell them apart (particularly in the laboratory where all we see is the sample and not the patient). They both will result in hemoglobinemia and ghost cells. However, if the animal is anemic and has hemoglobinuria, true intravascular hemolysis, i.e. a pathological hemolytic anemia, is likely. In the laboratory, we can also sometimes tell if hemolysis in plasma is an artifact. If we see pink or red plasma when examining plasma appearance as a part of a hemogram (collected into EDTA) but the serum or plasma in the chemistry panel shows no evidence of hemolysis (hemolytic index <20 units), in vitro hemolysis is likely. We see this frequently in mailed in samples in the dead of winter, where samples may freeze during shipping. We frequently see more hemolysis in serum samples than plasma samples, because RBCs are ruptured manually during removal of serum from the clot. With in vitro hemolysis, the RBC result that most accurately reflects the oxygen-carrying capacity is the hemoglobin (because the analyzer lyses the RBCs to measure hemoglobin, it does not matter if they were “prelysed” in the tube). Conversely, as described above, the hemoglobin may over-estimate the oxygen carrying capacity in a “true” in vivo hemolytic anemia. With both in vivio or in vitro hemolysis, the MCH and MCHC may be falsely increased (because hemoglobin is the common numerator for these calculated indices and is higher than the denominators, which are RBC count and HCT, respectively).
Finding | In vivo (true) | In vitro (artifactual) |
Hemoglobinemia in fresh sample | Yes | Unlikely – possible if poor sample collection |
Hemoglobinemia in stored sample | Yes | Yes |
Ghost cells | Likely | Likely |
Identifiable cause (e.g. oxidant) | Maybe | No |
Hemoglobinuria | Yes | No |
RBC result reflecting oxygen-carrying capacity | RBC count, HCT, PCV | Hgb |
Causes of intravascular hemolysis
- Immune-mediated hemolytic anemia: Complement fixation by IgG or IgM causes assembly of the membrane attack complex (MAC, C6-C9) on red blood cell membranes in vivo which lyses the cells. A variant of an immune-mediated hemolytic anemia is an acute hemolytic transfusion reaction where transfusion of incompatible blood into an animal will cause acute intravascular hemolysis when antibodies bind to the transfused “foreign” red blood cells and activate the complement cascade.
- Erythroparasites: Babesia species replicate inside erythrocytes and rupture the cells when they exit to continue their life cycle. This results in an intravascular hemolysis. Indeed, Babesia bovis infections are often called “red-water” disease due to the accompanying hemoglobinuria.
- Other infectious organisms: Specific bacteria that produce toxins that lyse RBC, such as Clostridium species and Leptospira, can cause lysis of RBC in vivo, either directly through the action of toxins or indirectly via inducing an immune-mediated anemia (Reef 1983, Weiss and Moritz 2003, Andersen et al 2013). There have been reports of bee stings (Noble and Armstrong 1999, Lewis and Racklyeft 2014) spider bites and snake venoms causing intravascular hemolysis (due to phospholipases in the venom) (Masserdotti 2009, Arce-Bejanaro et al 2014, Pagano et al 2016).
- Oxidant injury: Oxidant injury (e.g. copper toxicity in sheep [Solie and Froslie 1977] or dogs [Watson et al 1983], red maple or Pistacea toxicity in horses or camelids [Dewitt et al 2004, Alward et al 2006, Walter et al 2014], zinc toxicity in dogs from ingesting zinc pennies minted after 1982 [Latimer et al 1989, Meurs et al 1991]) can result in intravascular hemolysis.
- Metabolic conditions: Acute liver disease in horses can result in intravascular hemolysis (the mechanism is unknown, however increased bile acids, which can emulsify membranes, have been postulated as a cause). Because phosphate is essential for ATP production and maintenance of the integrity of red blood cell membranes, intravascular hemolysis can occur with severe hypophosphatemia (e.g. phosphate depleted dogs and cats with diabetes mellitus that are treated with insulin, post-partum hypophosphatemia in dairy cows). Water intoxication can also result in intravascular hemolysis.
- Inherited red blood cell defects: Dogs with phosphofructokinase deficiency (reported in English Springer Spaniel, American Cocker Spaniel and other breeds) can suffer from bouts of intravascular hemolysis with exercise, due to alkaline fragility of their red blood cells (Giger and Harvey 1987, Owen and Harvey 2012).
Intra- vs extravascular hemolysis
We use some laboratory features to identify when intravascular hemolysis is occurring with extravascular hemolysis, as summarized in the table below. As indicated above, extravascular hemolysis is always occurring and is usually seen alone (without intravascular hemolysis).
Finding | Intravascular | Extravascular |
Hemoglobinemia (serum or plasma should be red to some degree)* | Yes | No |
Hemoglobinuria (urine should be red to some degree, there is a positive reaction for heme but no RBC in sediment, normal CK, ruling out myoglobin as the cause for the heme reaction and red urine color) | Yes | No |
RBC morphologic features | Ghost cells (can be an artifact of smear preparation in any blood smear) | Other changes may be present, depending on cause |
RBC parasites | Babesia, Theileria | Any |
Total (usually indirect) bilirubin | Can be normal or increased (mostly due to the extravascular hemolysis that is present) | Normal or increased (if liver uptake/conjugation cannot keep up) |
Iron, percent saturation | Normal or increased | Normal or increased |
* Must differentiate from in vitro or artifactual hemorrhage (see table above). |
Hemorrhage vs hemolysis
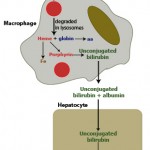
We use a variety of clinical and laboratory features to help us distinguish between these causes of a regenerative anemia. These include evaluation of the patient for evidence of hemorrhage (external hemorrhage can be far more obvious than internal hemorrhage, unless it is mild or intermittent), hematologic results (specifically, red blood cell morphologic changes compatible with hemolytic anemia if they are present in sufficient numbers), and chemistry results (specifically, protein, bilirubin and iron panel results). Note that additional tests may be required to document hemorrhage, e.g. fecal occult blood, peritoneal or pleural fluid analysis. A low total protein due to combined decreases in albumin and globulins is expected (but not inevitably present in animals with hemorrhage, particularly external, but also acute internal hemorrhage). However, other disease processes, e.g. concurrent inflammation, may affect results (inflammation may increase globulins as part of an acute phase or antigenic response). Total bilirubin concentrations can be, but are not always, increased in a hemolytic anemia because unconjugated bilirubin is produced from red blood cell breakdown in macrophages (extravascular hemolysis, see above). This will occur if production exceeds the ability of the liver to take up and conjugate the unconjugated bilirubin presented to it. Therefore, in an uncomplicated hemolytic anemia (most hemolytic anemias in ruminants and horses), unconjugated bilirubin dominates with minimal increases in conjugated (direct) bilirubin. In some small animal patients with hemolytic anemia (particularly dogs with immune-mediated hemolytic anemia), conjugated bilirubin may dominate because of concurrent cholestasis (mechanisms, unclear), however unconjugated bilirubin will still be increased. If the total bilirubin is high (> 5mg/dL), it is likely that both conjugated and unconjugated bilirubin will be increased in the dog and cat with hemolytic anemia, indicating concurrent cholestasis and hemolysis as a cause of the increased total bilirubin. Bilirubin would not be expected to be abnormal with hemorrhage, unless the animal has concurrent liver disease (always exceptions to any rule and very few “real” rules). With chronic external hemorrhage, iron is lost concurrently with RBC. Once iron stores are depleted, serum iron values (and percentage saturation of transferrin) will become decreased, however inflammation can also result in similar changes in iron and % saturation, decreasing specificity of these tests for iron deficiency. In hemolytic anemia, due to increased RBC (and iron) turnover, iron values can be high, along with % saturation. However, if the animal has concurrent inflammation, iron values (and % saturation) may be normal to even low. Ultimately, distinction between hemorrhage and hemolysis requires documentation of a source of bleeding and a decision by the clinician as to whether this is serious or severe enough to result in the anemia (frequently a judgement call). In some animals, identifying the exact mechanism of the anemia can be difficult and there may be multifactorial causes for anemia, e.g. a dog with disseminated intravascular coagulation can have hemorrhage due to coagulation factor and platelet defects and have a fragmentation hemolytic anemia. The table below summarizes these changes.
Finding | Hemorrhage | Hemolysis |
Evidence of bleeding (internal or external) severe enough to cause anemia | Yes | No |
Total protein (chemistry superior to refractometric measurement) | Usually low | Normal to high |
RBC morphologic changes (e.g. spherocytes) | No | Hopefully |
Total bilirubin (mostly indirect or unconjugated, exceptions given above) | Normal | Normal to increased |
Iron panel (iron and percentage saturation of transferrin) | Normal, low | Normal, high |
* There are always exceptions that are not listed in the table. |
Decreased production
Decreased or ineffective bone marrow production of erythroid cells will result in a non-regenerative anemia. Remember that, with acute hemorrhage or hemolysis, a regenerative response may not be seen for 3 to 5 days, especially if the anemia is mild to moderate, i.e. such anemias may initially appear non-regenerative. Mechanisms and causes of non-regenerative anemia have been reviewed (Grimes and Fry 2015).
Mechanisms of decreased production
- Insufficient production or activity of erythropoietic cytokines, e.g. chronic kidney disease (erythropoietin is produced in the kidney).
- Suppression of erythropoiesis: This can be mediated by drugs, cytokines (TNFα, interleukin-1, IFNγ), tumor cells or immune cells. Erythropoietic precursors can be directly or indirectly suppressed through inhibition of erythropoietin release or interference with its action on progenitors (usually due to inflammatory cytokines). Infiltrating tumors can also suppress erythropoiesis by “crowding out” marrow cells or competition for nutrients and minerals.
- Deficiency of minerals/vitamins/nutrients: The bone marrow needs fuel to do its work. Iron and copper essential for hemoglobin synthesis, whereas DNA synthesis requires folate and vitamin B12. Absence of these essential nutrients can result in macrocytic (vitamin B12, folate) or microcytic (iron, copper) anemias. General malnutrition can also result in anemia, which is usually normocytic normochromic. Sequestration of iron by inflammatory cytokines in various diseases (anemia of inflammatory disease) can also suppress erythropoiesis but the anemia is usually normocytic and normochromic versus an absolute iron deficiency (e.g. chronic external blood loss).
- Defective hemoglobin synthesis: This is usually secondary to iron deficiency and results in a microcytic hypochromic anemia (that may or may not be regenerative). Copper deficiency, zinc excess and inherited defects in hemoglobin synthesis (more common in humans) can produce a similar anemia.
- Defective DNA synthesis or nuclear maturation: Impairment of DNA synthesis characteristically results in macrocytic anemias. This can be due to dietary deficiency, drugs that interfere with DNA synthesis (e.g. hydroxyurea), inherited disorders of RBC production, and neoplasia (e.g. myelodysplastic syndrome).
- Destruction of bone marrow hematopoietic cells: This can affect RBC only or multiple hematopoietic lineages. It can be due to immune-mediated causes, drugs or toxins, neoplasia (which can efface the marrow or crowd out normal hematopoietic cells), infectious diseases, or ischemic injury (e.g. thrombosis causing bone marrow necrosis).
- Replacement of hematopoiesis: This is due to neoplasia (e.g. acute leukemia), which can efface the marrow or crowd out normal hematopoietic cells (called myelophthisis). This usually affects multiple hematopoietic lineages, resulting in bi- or pancytopenia.
Causes of decreased production
There are different causes of non-regenerative anemia, which may have overlapping mechanisms. Since marrow production is defective in non-regenerative anemia, a bone marrow aspirate may be indicated for specific diagnosis (or to narrow down the differential diagnostic list), determination of the underlying cause of the anemia, prognosis, and treatment. In general, a bone marrow is not indicated if the anemia is mild to moderate and the animal has a disease process that does not primarily involve the marrow, e.g. liver disease, renal disease. A marrow is indicated if the anemia is moderate to severe, other cytopenias (neutropenia and thrombocytopenia) are present, abnormal cells are detected in circulation, or there is no other explanation for the anemia. Note that many animals have anemia due to multifactorial causes (e.g. hemorrhage and decreased bone marrow production from inflammatory disease). In these cases, the anemia may be more severe than can be attributed to a single cause alone or the degree of regeneration is unexpectedly low for a hemorrhagic or hemolytic anemia.
Clues to help identify the cause of a non-regenerative anemia
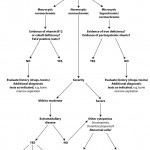
The following features are helpful to determine the cause of the non-regenerative anemia (see diagnostic algorithm):
- Clinical history: Drugs can affect bone marrow production of one or multiple cell lines, through various mechanisms (see above). Ensure that the anemia is not due to acute hemorrhage or hemolysis (with the bone marrow having insufficient time to mount a regenerative response).
- Presence of underlying disease: Many diseases that do not primary involve the bone marrow, (e.g. hepatopathy, nephropathy, cancer, endocrinopathy) may affect bone marrow production of RBCs. Naturally, if cancer directly involves the bone marrow (e.g. acute leukemia), the anemia may be quite severe and other cytopenias may be present. The most common cause of a normocytic normochromic non-regenerative anemia is the anemia of inflammatory disease.
- Severity of anemia: A mild to moderate anemia is usually due to suppression of erythropoiesis from inflammatory cytokines (anemia of inflammatory disease) or lack of erythropoietin (anemia of chronic renal failure, side-effect of exogenous human erythropoietin therapy). A more severe anemia suggests that the bone marrow is the main source of the anemia. Thus, a bone marrow aspirate is often needed for diagnosis of the cause of a severe non-regenerative anemia (particularly if extramedullary disease can be excluded). An example of a bone marrow disease that results in a severe anemia is non-regenerative immune-mediated anemia.
- Red blood cell indices: These can be helpful in determining cause.
- Normocytic normochromic anemia is the most common type of non-regenerative anemia and is usually due to inflammatory, renal or endocrine disease.
- Macrocytic anemias usually indicate a problem with DNA maturation and can occur with dietary deficiency (vitamin B12, folate, cobalt) or excess (e.g. molybdenum), drugs, infections (feline leukemia virus), neoplasia (e.g. myelodysplastic syndrome), and immune-mediated and inherited disorders. RBC can also swell when they imbibe fluid secondary to osmotic effects (e.g. diabetes mellitus). Note that some breeds of dogs, e.g. miniature and toy poodles, can normally have macrocytic RBC but are not anemic.
- Microcytic anemias usually indicate a problem with hemoglobin synthesis. This is mostly due to iron deficiency, but can also be caused by other dietary deficiencies (copper, vitamin B6) or excess (e.g. zinc), drugs, portosystemic shunts (in which there is altered iron metabolism – note that not all animals with shunts will be anemic but they are frequently microcytic), and immune-mediated and inherited disorders. Remember that young animals (< 2-3 months of age) can have a microcytic anemia due to age (physiologic iron deficiency combined with rapid growth). Also calves and foals can remain microcytic (but not anemic) up to 1 year of age and some breeds of dogs (e.g. Akitas) can normally have microcytic RBC but are not anemic.
- Other cytopenias: The presence of neutropenia (particularly with no left shift or toxic change) and/or thrombocytopenia supports a primary disease process in the bone marrow, which usually requires a bone marrow aspirate for diagnosis. Causes of multiple cytopenias (called bicytopenia or pancytopenia) are immune-mediated disorders, acute leukemia, infiltrative neoplasia (e.g. lymphoma), infections (e.g. Ehrlichia canis), and drugs. Note, when using blood to evaluate hematopoiesis, the most important cell types are RBC, neutrophils and platelets. The other leukocytes are long-lived cells and proliferate (monocytes, lymphocytes) hence their numbers are less affected by bone marrow disease.
- Abnormal cells: The presence of abnormal hematopoietic cells or immature cells (blasts) usually indicates primary bone marrow neoplasia, e.g. myelodysplastic syndrome or acute leukemia. Bone marrow aspiration is naturally indicated to confirm this.