Physiology
Creatine kinase (CK) is a “leakage” enzyme present in high concentrations in the cytoplasm of myocytes (skeletal and cardiac primarily) and brain and is the most widely used enzyme for evaluation of muscular disease. In muscles, CK functions by making ATP available for contraction by phosphorylating ADP from phosphocreatine. Phosphocreatine is the major storage form of high energy phosphate in muscle. Creatine kinase is a dimeric molecule composed of two types of monomers, the M and B subunits. Various combinations of these monomers make up different CK isoenzymes which are found in specific locations in brains and cells (see below). The routine activity assay measures total CK activity and not the individual isoenzymes. Isoenzymes may be quantitated by electrophoretic or immunologic methods. This is most commonly used in humans with ischemic cardiac events (heart attack) but has been supplanted by measurement of cardiac troponin in many hospitals. Due to the difficulties in identifying CK isoenzymes in animals and the perceived scarcity of cardiac injury, we do not quantitate the different isoenzymes. In addition, we use troponin as a marker of cardiac injury in animals. There are four principle isoenzymes of CK:
- CK-1: BB isoenzyme, found mostly in the brain. Injury to brain tissue may increase CK-1 activity in CSF, but rarely results in raised total serum CK activity.
- CK-2: MB isoenzyme, highest concentration in cardiac muscle, with lesser amounts in skeletal muscle.
- CK-3: MM isoenzyme, found in skeletal muscle, with lesser amounts in cardiac muscle. This isoenzyme accounts for the majority of CK in plasma of animals.
- CK-Mt: Found in mitochondrial membranes, and comprises 15% of total cardiac CK activity. There are ubiquitous and sarcomeric forms of CK-Mt.
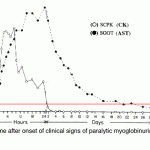
CK can also be found in macroforms. For example, macro-CK1 is dimeric CK1 bound to immunoglobulin.
In one study, CK isoenzymes were measured in normal dogs (n=20) and cats (n=14) by electrophoresis. The dominant isoenzyme was CK-MM (CK-3) in both species. In dogs, both macroCK-2 and CK-BB (CK-1) were the next most frequent forms of CK, whereas in cats both macroenzymes were the second most frequent form. Increases of CK-BB were seen in 3 dogs with central neurologic disease (Paltrinieri et al 2010).
Creatine kinase has a very short half life. In horses, intravenous infusion of a muscle homogenate revealed a terminal half life of 123 minutes (mean) (Volfinger et al 1994). In dogs, the CK half life is 2.5 hours after intravenous administration of a muscle homogenate and 6.5 hours after intramuscular administration of the said homogenate (Aktas et al 1995). Activity increases quickly (increases at 5 hours and peaks within 12 hours) and returns to normal within 24-48 hours after acute, transient muscle injury in horses. Persistent or ongoing muscle injury will maintain high CK activity. In contrast, aspartate aminotransferase (AST) (which has a longer half-life of 24 hours in the dogs and several days in the horse) will increase more gradually after muscle injury and stays increased for a longer period of time than CK (see image).
Methodology
The routinely used enzymatic assay takes advantage of the normal ability of CK to catalyze the conversion of phosphocreatine to creatine, releasing ATP in the process.
Reaction type
Kinetic with UV measurement
Procedure
Nicotinamide adenine dinucleotide phosphate (NADPH) and ATP are produced in equal quantities at the same rate. The increase in NADPH formation is directly proportional to the CK activity.
Creatine phosphate + ADP CK > creatine + ATP
Glucose + ATP Hexokinase > Glucose-6-P + ADP
Glucose-6-P + NADP+ G6P-DH > Gluconate-6-P + NADPH + H+
Units of measurement
Creatine kinase activity is measured in U/L (conventional units) and µkat/L (SI units), which is defined as the amount of enzyme that catalyzes the conversion of 1 µmol of substrate per minute under specified conditions. The conversion formula is shown below:
U/L x 0.0167 = µkat/L
Sample considerations
Sample type
Serum, plasma, cerebrospinal fluid.
Anticoagulant
Heparin (lithium) or EDTA
Stability
According to the reagent manufacturer, stability of CK in human serum and plasma samples is as follows: 2 days at room temperature, 7 days refrigerated, or 4 weeks frozen at around -20°C.
Interferences
- Lipemia/turbidity: Severe turbidity (>1000 turbidity index) may increase concentrations.
- Hemolysis: May mildly increase with moderate hemolysis (>100 hemolysis index). This is due to the reasons indicated below.
- Icterus: Severe icterus may increase activity (>60 icteric index)
Test interpretation
Increased concentration/activity
Increases in serum or plasma CK is most commonly used as a marker of skeletal muscle injury (by sheer mass, it is unlikely to see increases in total CK activity in serum or plasma after cardiac injury, however increases in CK-MB or CK-2 may be seen if electrophoretic separation is done).
- Artifact: Moderate hemolysis may increase CK activity as constituents within red blood cells (ADP or glucose-6-phosphate) or in their membranes (adenylate kinase) contribute to the enzymatic reaction for CK, falsely increasing activity. Inadvertent penetration of muscle (“muscle stick”) during venipuncture can cause 3- to 4-fold increases in CK activity in the sample and is a common cause of mildly increased CK values in healthy (or sick) animals. We have seen that CK activity can be as high as 3-4000 U/L from a muscle stick (which we consider a mild increase).
- Physiologic: CK activity in young puppies is higher than in adults, although this is apparently without explanation. Moderate increases (2-3x) are possible in exercising horses, with the post-exercise increase being less in well-conditioned animals. Intramuscular injections will increase CK activity to various degrees depending on the irritant or toxic effects of the drug or if the drug impedes local blood flow.
- Pathophysiologic
- Muscle disease: Detection of increased activity in serum is useful as an indicator of muscle injury. The assay is quite sensitive in this regard, but increased activity is not specific as to cause (e.g., trauma, inflammation, degeneration). High CK activity are observed in inherited muscular dystrophies, exercise-induced rhabdomyolysis, polymyositis, vitamin E-selenium deficiency, snake bite poisoning, etc. In one study, increased CK activity was seen in 60% of sick cats with various diseases, with marked increases (>30x upper reference limit) only being seen in 7% of cats. Some of these increases could potentially be due to inadvertent muscle sticks (Aroch et al 2010). Note that animals which are recumbent (“downer” cows or post-surgical patients) will have high CK activity (up to 10 x normal) from muscle injury or ischemia. Similarly, horses and cattle after shipping have moderate increases in CK activity.
- Rhabdomyolysis: Severe muscle injury releases myoglobin, which is nephrotoxic. Many patients with acute severe rhabdomyolysis are at risk of renal failure, particularly if there is concurrent hypovolemia and aciduria. The renal injury is attributed to renal hypoxia by myoglobin-scavenging the vasodilator nitric oxide or stimulating vasoconstrictor release, e.g endothelin. The iron component within myoglobin acts as a free radicle, inducing direct injury to the renal tubular cells (e.g. causes lipid peroxidation), and myoglobin precipitates into casts with Tamm-Horsfall protein, particularly at low pH, causing tubular obstruction and severe renal damage. Damaged muscles not only release myoglobin, they also release potassium, phosphate, and urate. Calcium movement intracellularly can exacerbate the muscle injury (Huerta-Alardin et al 2005).
- Exercise-induced rhabdomyolysis in horses: This is a genetic trait in Quarterhorses, who develop a severe myopathy with myoglobinuria and potential secondary renal toxicity. The trait has been linked to a missense mutation E32G1) in a gene encoding for one of the heavy chains (2X) of myosin (MYH1) (Valberg et al 2018). Other breeds also suffer from exertional rhabdomyolysis, including Arabians and Andalusians and the underlying pathogenesis varies (Chamizo et al 2015, Valberg et al 2016).
- Immune-mediated polymyositis: This occurs in various species, including dogs and horses. In Quarterhorses, a lymphocytic myositis has been associated with the same genetic mutation as that seen in horses with exercise-induced myositis (Finno et al 2018, Valberg et al 2018).
- Rhabdomyolysis: Severe muscle injury releases myoglobin, which is nephrotoxic. Many patients with acute severe rhabdomyolysis are at risk of renal failure, particularly if there is concurrent hypovolemia and aciduria. The renal injury is attributed to renal hypoxia by myoglobin-scavenging the vasodilator nitric oxide or stimulating vasoconstrictor release, e.g endothelin. The iron component within myoglobin acts as a free radicle, inducing direct injury to the renal tubular cells (e.g. causes lipid peroxidation), and myoglobin precipitates into casts with Tamm-Horsfall protein, particularly at low pH, causing tubular obstruction and severe renal damage. Damaged muscles not only release myoglobin, they also release potassium, phosphate, and urate. Calcium movement intracellularly can exacerbate the muscle injury (Huerta-Alardin et al 2005).
- Miscellaneous: Critically ill anorectic cats with various diseases, including cholangiohepatitis, vestibular disease, hepatic lipidosis, chronic renal disease, septicemia, hyperthyroidism, and renal disease (chronic kidney disease, pyelonephritis) had higher median CK activity than clinically healthy cats or non-anorectic cats with minor diseases. The anorectic cats had a median CK activity of 2500 U/L (range, 88 to 153,000/uL) with the highest activities seen inc cats with insulin overdose, septicemia, lipidosis. and hyperthyroidism. The CK activity was moderately positively associated with AST (correlation of 0.59) and LDH (correlation of 0.41), increases of which can also be associated with muscle injury (Fascetti et al 1997). The increases were postulated to be due to increased muscle catabolism or membrane permeability (blood was withdrawn through an indwelling catheter in these cats, arguing against a muscle puncture during collection). It is possible that the muscle was secondary to the primary disease process versus anorexia per se. Furthermore, this study lacked a suitable control group of ill but not anorectic cats. The CK did decline with disease resolution however this argues more for the natural short half life of CK versus a consequence of resolution of the anorexia.
- Neurologic disease: Creatine kinase activity has been measured in the cerebrospinal fluid of animals as a marker of neurologic injury. Studies in horses have shown that high CK activity is seen in various neurologic conditions, including equine protozoal neuritis (Sarcocystis neurona) but that high values are neither sensitive or specific for the latter disease. Furthermore, contamination with epidural fat is a major confounding variable that falsely increases CK activity (Jackson et al 1996).
- Muscle disease: Detection of increased activity in serum is useful as an indicator of muscle injury. The assay is quite sensitive in this regard, but increased activity is not specific as to cause (e.g., trauma, inflammation, degeneration). High CK activity are observed in inherited muscular dystrophies, exercise-induced rhabdomyolysis, polymyositis, vitamin E-selenium deficiency, snake bite poisoning, etc. In one study, increased CK activity was seen in 60% of sick cats with various diseases, with marked increases (>30x upper reference limit) only being seen in 7% of cats. Some of these increases could potentially be due to inadvertent muscle sticks (Aroch et al 2010). Note that animals which are recumbent (“downer” cows or post-surgical patients) will have high CK activity (up to 10 x normal) from muscle injury or ischemia. Similarly, horses and cattle after shipping have moderate increases in CK activity.