Triglycerides (TG) are lipid compounds consisting of a glycerol (a 3-carbon molecule containing three hydroxyl groups) backbone esterified to three long chain fatty acids. Triglycerides are a vital energy source for cells. Circulation of triglycerides through the vasculature is achieved by the incorporation of these compounds into lipoproteins. Lipoproteins are composed of a coat of phospholipid, cholesterol and proteins (apolipoproteins) enclosing a hydrophobic center of cholesterol esters and triglycerides. There are four classes of lipoproteins, high-density lipoproteins (HDL), low-density lipoproteins (LDL), very low-density lipoproteins (VLDL) and chylomicrons (CM). All these lipoprotein classes contain TG, however the highest triglyceride concentrations are found in CM and VLDL. Lipoproteins shuttle triglycerides from intestines (ingested or “exogenous” TG or CM) and liver (synthesized or “endogenous” triglycerides from NEFA) to cells for energy or adipose tissue for storage. They also shuttle cholesterol to tissues (LDL) and liver (HDL) for use. So triglycerides are coming from:
- Diet: Absorbed as CM. Will result in a visible lipemia in dogs and cats after eating, but not horses or ruminants (who eat all the time). Broken down by lipoprotein lipase (LPL) and remnants taken up by liver and broken down to constituents for use.
- Liver: Synthesized from non-esterified fatty acids (NEFA) by adding glycerol to three NEFAs. NEFAs can come from various sources, including broken down chylomicron remnants and lipolysis. The triglycerides are then packaged into VLDL, which is done better in some species than others. Cattle are poor VLDL producers, so they have to do something else with their triglycerides and NEFAs (when in excess) so they store triglycerides in the liver (which can lead to hepatic lipidosis) and convert NEFA to ketones (leading to subclinical and clinical ketosis).
An increase in triglyceride in serum or plasma as measured on a chemistry panel is usually due to an increase in chylomicrons or VLDL, but very high concentrations of the other lipoproteins may also increase triglycerides, although cholesterol increases will be much higher. Post-prandial or pathologic increases in CM in dogs and cats can result in visible lipemia (usually with a fat layer), but this is not expected in other species. Pathologic increases in VLDL can result in visible lipemia (cloudy infranatant, no fat layer) in dogs and cats (if concentrations are high enough) and in camelids, but is rarely seen in horses and not in cattle.
Synonyms
Triacylglycerol
Physiology
The concentration of triglycerides-rich lipoproteins (CM, VLDL, and IDL) in blood is controlled by a variety of hormones. Insulin, insulin antagonists, and other hormones affect lipoprotein concentrations by activating or inhibiting the activities of lipoprotein lipase, hepatic lipase, and hormone-sensitive triglyceride lipase. These hormone regulated enzymes stimulate metabolic processes such as lipolysis and hepatocyte clearance of lipoprotein remnants. Disorders disrupting CM and VLDL metabolism result in hyperlipoproteinemia (↑ [lipoprotein]) thus leading to hypertriglyceridemia (↑ [triglyceride]). Mechanisms of the enzymes involved in lipoprotein metabolism are described below:
- Lipoprotein lipase (LPL): Lipoprotein lipase is found in the vascular endothelium. It is activated by insulin, ACTH, TSH, glucagon and thyroid hormone. Its activity is enhanced by heparin. Lipoprotein lipase hydrolyzes CM and VLDL to free (non-esterified) fatty acids and glycerol for tissue use. Cholesterol-rich remnants produced from both lipoproteins are removed by the liver. Lipoprotein lipase is one of the several enzymes that also converts VLDL to LDL (via IDL). Apolipoprotein C-II is essential for activation of LPL.
- Pancreatic lipase: Pancreatic lipase degrades dietary fat in the gastrointestinal lumen, allowing uptake and packaging into CM.
- Hepatic lipase: This enzyme hydrolyzes surface phospholipids on lipoproteins and is responsible for removing triglycerides from LDL (and helps the liver remove LDL from circulation).
- Hormone-sensitive triglyceride lipase: This enzyme is responsible for lipolysis (mobilization of triglycerides from adipose tissue to yield non-esterified fatty acids [NEFA] and glycerol). The enzyme is stimulated by catecholamines, glucagon, growth hormone, thyroxine, ACTH, corticosteroids and prostaglandins. It is inhibited by insulin (or the hormone is most active in the absence of insulin). Liberated NEFAs are transported to the liver (free or albumin-bound), where they are taken up and used for energy (β-oxidation), re-combined with glycerol to form VLDL, or incorporated into ketones. Therefore, lipolysis will increase VLDL production.
Methodology
Reaction type
End-point reaction
Procedure
This is based on disruption of triglycerides by lipoprotein lipase, which breaks it apart into NEFAs and glycerol. The assay measures the glycerol component (NEFAs are a separate measurement entirely).
- Triglycerides GPO-PAP method: In the first step of this 4-stage reaction, the enzyme lipoprotein lipase (LPL) catalyzes the complete hydrolysis of triglycerides yielding glycerol and fatty acids. Step 2 involves the enzyme glycerokinase (GK) which, in the presence of Mg2+, catalyzes the phosporylation of glycerol from ATP. In the following step, glycerophosphate oxidase (GPO) catalyzes the oxidation of glycerol-3-phosphate, produced in the subsequent reaction, to dihydroxyacetone phosphate and hydrogen peroxide. Finally, under the catalytic action of peroxidase hydrogen peroxide reacts with 4-aminophenazone and 4-cholorophenol to form the red dye product 4-(p-benzoquinone-monoimino)-phenazone. The color intensity of the red dye product is measured photometrically, at 500 nm, and is directly proportional to the triglyceride concentration (reported in mg/dL)
- Reactions are shown below:
triglycerides + 3H2O lipoprotein lipase > glycerol + fatty acids
glycerol + ATP glycerol kinase > glycerol-3-phosphate + ADP
glycerol-3-phosphate + O2 glycerol-3-phosphate oxidase> dihydroxyacetone phosphate + H2O2
H2O2 + 4-aminophenazone + 4-chlorophenol peroxidase > red-colored complex
- Lipoprotein quantification: At Cornell University, lipoprotein classes can be subfractionated using polyacrylamide gel electrophoresis (Lipoprint, Quantimetrix), although the triglyceride content of each subfraction is not known (Behling-Kelly et al 2022).
Units of measurement
Serum or plasma triglyceride concentration is measured in mg/dL (conventional units) or mmol/L (SI units). The conversion formula is shown below:
mg/dL x 0.011 = mmol/L
Sample considerations
Sample type
Serum, plasma, body cavity fluids (peritoneal, pleural)
Anticoagulants
Heparin or EDTA
Stability
Triglyceride concentrations in serum are stable for variable periods of time within a range of cold temperatures in humans: 10 days at 2-8 °C, 3 months frozen at -20°C, and several years frozen at -70°C (per product information sheet). To maintain the highest attainable level of stability avoid repeated thawing and freezing cycles.
Interferences
- Lipemia : None of these interferents affect the triglyceride concentration substantially with the methods used by Cornell University. Note, that the term (result) lipemic index implies it is an assessment of triglyceride concentrations, the lipemic index in reality does not correlate well to triglyceride concentrations and is more of a reflection of sample turbidity.
- Icterus: Can falsely decrease concentrations (icteric index > 9).
- Hemolysis: No interference up to a hemolytic index of 700 (marked hemolysis).
- Drugs: No interference was observed using common drug panels.
Test interpretation
Low triglyceride concentrations are not of diagnostic relevance.
Increased concentration (hypertriglyceridemia)
In dogs and cats, hypertriglyceridemia is commonly due to a physiologic change that occurs after eating. Hypertriglyceridemia in the fasting state usually reflects abnormal lipoprotein metabolism, but can be seen in low numbers of clinically healthy animals (Yi et al 2022).
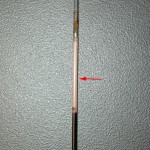
- Physiologic or post-prandial: Hypertriglyceridemia after eating is usually due to increased chylomicrons, which are large particles that disperse light and cause gross lipemia. A post-prandial hypertriglyceridemia is seen in dogs and cats. Chylomicrons are produced in the intestine after absorption of dietary (exogenous) fat. Lipemia interferes with the measurement of many clinical pathology analytes so it is important to withhold food prior to taking blood samples for analysis. Lipemia should clear within 4-6 hours after eating (12 hour fast recommended). In a study of 100 clinically healthy dogs, triglyceride concentrations increased in 92 dogs between 2-8 hours of eating with the peak median concentration at 2 hours after eating, with 36 dogs having values above the reference interval (Yi et al 2020).
- Drugs: Exogenous immunosuppressive or anti-inflammatory doses of corticosteroids can result in a fasting hypertriglyceridemia in dogs and cats (Lowe et al 2008, Khelik et al 2019, Tinklenberg et al 2020). This is attributed to inhibition of insulin action on lipoprotein lipase (increase CM and VLDL) and stimulation of hormone-sensitive lipase (causing lipolysis). The increase will be due to CM and VLDL (lipoprotein lipase clears both from blood).
- Pathophysiologic: A fasting hypertriglyceridemia usually indicates underlying defects in lipoprotein metabolism and is typically due to increased VLDL (endogenous lipid production), although CM could still persist for longer than expected if lipoprotein lipase activity is reduced (e.g. lack of or resistance to insulin). The hypertriglyceridemia may result in visible lipemia, particularly when due to chylomicrons or if VLDL concentratons are very high. In dogs, the most common causes of a fasting or pathologic lipemia are diabetes mellitus, hyperadrenocorticism, pancreatitis, and corticosteroid therapy. In horses, hypertriglyceridemia is usually due to metabolic syndrome, pituitary pars intermedia dysfunction (PPID, used to be called equine Cushing’s disease), and excess negative energy balance (ponies, donkeys). Visible lipemia can be seen in states of negative energy balance in ponies and donkeys (and other rare conditions) and is not a feature of equine metabolic syndrome or PPID, despite hypertriglyceridemia in these conditions. In camelids, excess negative energy balance (with associated hepatic lipidosis) is a cause of hypertriglyceridemia and lipemia (usually due to VLDL Increases). In contrast, high triglyceride concentrations and visible lipemia are not seen in cattle with excess negative energy balance. This may be because cattle do not produce many VLDL in response to lipolysis, but rather shift the liberated NEFA from lipolysis to ketone production (such as β-hydroxybutyrate) or fat deposition (lipidosis) in the liver instead.
- Inflammation: Dogs with septic inflammation have higher triglyceride concentrations than dogs with non-septic inflammation in one study, although triglyceride concentrations did not effectively discriminate between non-septic and septic inflammation. Quantification of lipoprotein subfractions i septic dogs showed a decrease in HDL and increase in LDL with the Lipoprint assay, although the triglyceride content of the fractions are not known. The cause for the increase in triglycerides in septic dogs is unclear (Behling-Kelly et al 2022). Diabetes mellitus: Hyperlipemia is due to a marked increase in triglycerides, with a concurrent mild to moderate increase in cholesterol. There is increased CM, VLDL and LDL. This is from a deficiency or lack of insulin, resulting in decreased activation of lipoprotein lipase (decreases CM and VLDL hydrolysis), increased lipolysis (enhances VLDL production) and downregulation of LDL receptors (increases LDL).
- Hyperadrenocorticism: Corticosteroids inhibit the action of insulin, resulting in decreased activity of lipoprotein lipase (clears VLDL and CM). Corticosteroids also promote lipolysis by stimulating hormone-sensitive lipase, which will increase VLDL production. Similar to diabetes mellitus, increases in cholesterol concentration can be seen with high VLDL concentrations.
- Pancreatitis: Hyperlipemia is mostly associated with hypertriglyceridemia with a mild increase in cholesterol. This is attributed to increased CM and VLDL from decreased lipoprotein lipase activity.
- Excessive negative energy balance: In states of excessive negative energy balance (e.g. starvation, anorexia) particularly when energy demands are high (e.g. late pregnancy, early lactation), lipolysis of fat stores in adipocytes will increase VLDL concentrations. Hyperlipemia due to excessive negative energy balance mostly occurs in ponies, donkeys and camelids and is due to increased energy demands from lactation or pregnancy combined with insufficient food intake (stress, transport, underlying disease), insufficient dietary energy or insulin resistance (from pregnancy or stress-associated hormones). In contrast, ruminants with excessive negative energy balance rarely develop triglyceride or cholesterol abnormalities (which has been attributed to inefficient export of VLDL by the liver in these species). In all species, excessive negative energy balance can cause hepatic lipidosis (non-esterified fatty acids from lipolysis are converted to triglycerides and are stored, when in excess, as fat in hepatocytes).
- Hyperlipemia syndrome in horses: This is especially seen in pony mares and donkeys and is associated with obesity, pregnancy, stress (e.g. transport) and lactation. It can also occur in miniature horses with gastrointestinal conditions, such as enterocolitis (Mogg and Palmer 1995). Hyperlipemic syndrome is characterized by negative energy balance (resulting in lipolysis) and insulin resistance (from pregnancy-associated hormones like progesterone and obesity). Horses have poorly developed pathways for ketone production and hence cannot convert mobilized fatty acids to ketone bodies, shifting them instead to VLDL production. Hypertriglyceridemia is due to increased VLDL and results in hepatic lipidosis (and eventually liver failure with increased liver enzymes), hypoglycemia, renal disease and central nervous system signs. It is fatal in more than 60% of cases. Other diseases associated with hypertriglyceridemia in horses are pars pituitary intermedia dysfunction (hyperglycemia, insulin resistance, and hirsutism are characteristic, although some horses can develop hyperlipemia [Dunkel et al 2014]), insulin resistance due to metabolic syndrome (Geor and Frank 2009), excess negative energy balance (e.g. secondary to gastrointestinal conditions, such as colitis) and, rarely, cancer such as lymphoma (internal observations at Cornell). These diseases are seen in various breeds of horses, including Arabians (Waitt and Cebra 2009).
- Hyperlipemia in camelids: Camelids, unlike horses, can also suffer from ketosis when they are in excess negative energy balance. Pregnancy toxemia is the term used to refer to pregnant camelids with hyperlipemia (due to increases in triglycerides [mostly VLDL] especially, but also cholesterol does increase) and ketosis. Like horses, affected camelids often have underlying hepatic lipidosis and can die from their disease
- Familial hyperlipidemias: These are usually due to defects in lipoprotein lipase and have been reported in dogs (Miniature Schnauzers, Beagles, Brittany Spaniels) and cats (inherited hyperchylomicronemia in Siamese, domestic shorthair and Himalayans).
- Hypertriglyceridemia in Miniature Schnauzers is seen in middle aged or older dogs (> 4 years old). They have marked hypertriglyceridemia (triglycerides of 500-800 mg/dL) with increased VLDL and CM. Many have concurrent diabetes mellitus or pancreatitis.
- Inherited hyperchylomicronemia affects young cats (8-9 months old) and is associated with lethargy, inappetance, anemia, xanthomata (lipid granulomas in the skin and internal organs), neuropathy, and, if triglyceride concentrations are > 1500 mg/dL, lipemia retinalis. The disease is due to an autosomal recessive defect in lipoprotein lipase.