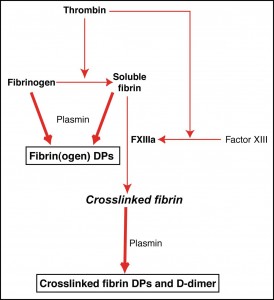
Tests for fibrinolysis involve evaluation of the fibrinolytic pathway. Unfortunately, measurement of many components of the fibrinolytic pathway, including plasminogen, tissue plasminogen activator, plasminogen activator inhibitor, have not been developed or validated in animals, or those that have, work poorly. Therefore, these tests are not offered routinely, which is a major shortcoming when evaluating animals for hemostatic or thrombotic disorders. The most common tests of fibrinolytic activity are the tests for fibrin(ogen) degradation products, including D-dimer.
Other tests that are not commonly used are the clot lysis time and the euglobulin lysis time. The clot lysis time is the time required for whole blood clots to lyse at 37 ºC (and can be performed after the clot retraction time) and is dependent on plasmin activity, fibrinogen concentration and the degree of clot retraction. Poor clot retraction will impede the lysis time. The clot is usually lysed within 8 to 20 hours in animals. The euglobulin lysis time is a measure of plasmin activity. In this test, euglobulins (fibrinogen, plasminogen, plasmin, plasminogen activator) precipitate on dilution with water, whereas fibrinolytic inhibitors (plasminogen activator inhibitor, antiplasmin) do not. The removal of these inhibitors allows the plasmin in the sample to lyse the precipitate with the time taken for lysis being the euglobulin lysis time. This test is quite crude and is not offered by most laboratories.
Fibrin(ogen) degradation products (FDP)
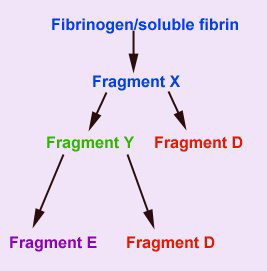
This assay detects the presence of circulating fragments (FDPs) of fibrinogen and soluble (non-crosslinked) fibrin that are produced by the action of plasmin on these substrates. Plasmin acts on these two substrates similarly, producing an initial cleavage product called fragment X. Plasmin then acts on fragment X, cleaving it into a transient fragment Y, and fragment D. Further cleavage of fragment Y, produces the terminal fibrin(ogen) degradation products, fragment D and E. Thus from one molecule of fibrinogen or soluble fibrin, two terminal FDP fragments D and one terminal fragment E is produced.
There are assays for FDPs in both serum and plasma. Serum FDP assays use polyclonal antibodies that cross-react with intact fibrinogen (that has not been lysed by plasmin), necessitating its removal. This is accomplished by the use of specialized collection tubes containing Botrox atrox venom (Reptilase) and inhibitors of fibrinolysis (aprotonin or soyabean trypsin inhibitor). All serum FDP assays use latex beads that are coated with antibody raised against human fibrin(ogen) degradation products, usually fragments D and E. The antibodies cross-react well enough with the fibrin(ogen) fragments of animals to make the test useful for veterinary patients. 2 mLs (as little as 0.5 mls can be used if the patient is very small) of blood from the patient is put immediately into one of the tubes provided with the test kit.
Serum from the tube is diluted 1:5 and 1:20. A small volume of each dilution is mixed on a plate with an equal volume of the antibody-coated latex beads. Positive and negative control sera are assayed concurrently. Agglutination of the beads at the 1:5 dilution indicates an FDP concentration of 10-40 µl/mL, whereas agglutination at both 1:5 and 1:20 dilutions indicates an FDP concentration >40 µl/mL. Either positive result is abnormal and indicates a higher than normal rate of degradation of fibrinogen and/or fibrin by plasmin or decreased clearance of these products.
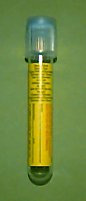
There are newer latex-agglutination kits that are based on monoclonal antibodies that do not cross-react with intact fibrinogen and can thus be used on citrated plasma samples. This is advantageous compared to serum FDP assays, as a specialized collection tube for FDP assay is not required and a single citrated blood sample can be used for all coagulation tests. This is the procedure performed by the Clinical Pathology laboratory at Cornell University and it has only been validated for the dog. The plasma FDP assay is performed similarly to the serum FDP assay, except dilutions of 1:2 and 1:8 are made, with results being reported as < 5 µl/mL, 5-20 µl/mL and > 20 µl/mL. Results > 5 µl/mL are abnormal.
The FDP assay is used with the other coagulation tests to characterize bleeding disorders more completely. The results should never be interpreted alone, without evaluation of clinical signs and results of other coagulation tests. Any cause of pathologic intravascular coagulation (the most common of which is DIC), thrombosis, or severe internal hemorrhage can produce FDP’s. In addition, any condition causing decreased clearance of FDPs by the liver and monocyte-macrophage system, e.g. severe liver disease, can also result in increased FDP values. A negative FDP result does not rule out these processes.
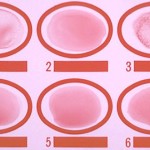
Note that coagulation can also occur in extravascular tissue spaces and high FDPs have been associated with fibrin(ogen)olysis occurring in protein-rich or hemorrhagic effusions into body cavities. Indeed, horses with colic due to gastrointestinal disorders have higher FDPs in blood and peritoneal fluid than healthy control horses. Although the high FDPs in the blood of these colic horses are compatible with DIC (which is often subclinical in horses and results in thrombosis rather than hemorrhage), the FDP values in their peritoneal fluid were higher than in blood, suggesting extravascular production of the FDPs (probably secondary to inflammation-induced tissue factor expression and fibrinolysis in the peritoneal cavity). This could have partly contributed to the high blood FDPs.
The serum FDP assay is of little use when evaluating horses for coagulopathies because of the high frequency of false positive results. Sera from most horses, whether healthy or ill, give positive tests with this assay. A single plasma assay has been evaluated and does not appear to crossreact with equine FDPs (all these latex agglutination assays use antibodies raised against human FDPs).
D-dimer
D-dimer is a specific plasmin-mediated breakdown product of crosslinked fibrin. Thrombin converts fibrinogen into soluble fibrin monomer. This monomer then spontaneously polymerizes to form the soluble fibrin polymer. Thrombin also activates factor XIII, which in the presence of calcium, crosslinks the fibrin polymer, producing crosslinked fibrin. Plasmin cleavage of fibrinogen or soluble fibrin produces the “traditional” FDPs, fragments X, Y, D and E. Plasmin cleavage of crosslinked fibrin produces different degradation products, that vary in molecular weight and are called X-oligomers. D-dimer is a specific neoantigen produced by the factor XIIIa-mediated crosslinking of fibrin and is exposed after plasmin degrades crosslinked fibrin, allowing it to be detected by immunologic-based assays. Note that although plasmin is the main fibrinolytic enzyme, proteolytic enzymes released by neutrophils can also degrade crosslinked fibrin exposing D-dimer.
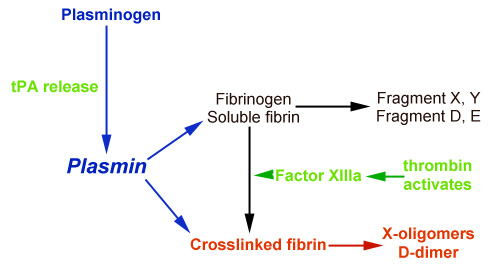
Thus, D-dimer is more specific for fibrinolysis than FDPs, as its formation requires the action of thrombin (to activate factor XIII) to produce crosslinked fibrin and the cleavage of this fibrin by plasmin. In contrast, traditional FDP assays cannot distinguish between plasmin action on fibrinogen (fibrinogenolysis) and fibrin (fibrinolysis), therefore FDPs can be elevated when there is no clot present (and plasmin is just cleaving fibrinogen).
D-dimer can be detected in human patients with assays using monoclonal antibodies specific for the human D-dimer epitope. Some of these monoclonal antibodies crossreact with some animal species and can be used for veterinary patients. Certain D-dimer latex agglutination assay have been validated in the dog, cat and horse. The test is run similarly to the FDP assays, but the sample can be assayed undiluted (to obtain a positive or negative result) or can be serially diluted to obtain a semi-quantitative D-dimer value. It is far better to get a semi-quantitative D-dimer result, because higher values may be more specific for thrombembolic conditions.
Normal values
Normal dogs and cats have D-dimer values < 250 ng/mL whereas most healthy horses have D-dimer values < 500 ng/ml (but D-dimer can be as high as 1000 ng/mL in the horse).
Increased D-dimer
D-dimer will be increased whenever there is activation of thrombin, to form crosslinked fibrin and fibrinolysis, i.e. thrombosis and fibrinolysis. The prototypical thromboembolic disease is disseminated intravascular coagulation (DIC) and D-dimer is often very high in this disorder (indeed, D-dimer is quite sensitive for DIC and values may increase in early DIC before any other coagulation assays, such as the PT and APTT, become abnormal). However, any disorder resulting in crosslinked fibrin formation and breakdown can potentially increase D-dimer concentrations (i.e. high D-dimer is not specific for DIC). This includes physiologic and pathologic fibrinolysis (associated with thrombosis of any cause, e.g. pulmonary thromboembolism).
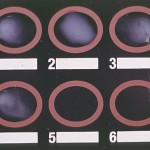
It should be noted that coagulation and fibrinolysis are not restricted to the intravascular space. If thrombin is activated in extravascular tissues in the presence of fibrinogen, e.g. protein-rich or hemorrhagic effusions into body cavities (e.g. joints, thoracic cavity, central nervous system), crosslinked fibrin could form in these extravascular sites (Andreassen et al 2017). Activation of plasmin (e.g. via urokinase plasminogen activator) or release of proteolytic enzymes from neutrophils within these sites could degrade this crosslinked fibrin, releasing D-dimer into the fluid. This could potentially be reabsorbed into blood, increasing plasma D-dimer levels. A study in dogs with various causes of ascites has shown that D-dimer concentrations are increased in dogs with transudates from decreased colloidal osmotic pressure, post-sinusoidal hypertension (e.g. cardiac disease), exudates (e.g. septic peritonitis, cancer-associated), and hemorrhagic effusions (e.g. hemangiosarcoma, anticoagulant rodenticide toxicity), with the highest median D-dimer concentration being seen in the fluid with the latter two causes. D-dimer concentrations were higher in the abdominal fluid than in the corresponding plasma, supporting extravascular fibrinolysis within the peritoneal cavity. This was despite lower median concentrations of fibrinogen in the abdominal cavity than in plasma (naturally dogs with exudative effusions had the highest median fibrinogen concentration in the ascitic fluid) (Zoia et al 2017). In addition, in DIC, D-dimer could be produced within extravascular spaces, secondary to endothelial dysfunction with leakage of large coagulation factors into the extravascular space (Thachil 2021).
Species-specific interpretation
- Dogs: Plasma D-dimer concentrations are often very high (> 1000 ng/mL) in dogs with documented thromboembolic disease, including DIC, or thrombosis (e.g. pulmonary thromboembolism with no laboratory evidence of DIC [Epstein et al 2013]). Indeed, D-dimer appears to be a very sensitive indicator of fibrinolysis in dogs with DIC (85-100% sensitive in one study [Stokol et al 2000]). However, D-dimer is not specific for DIC or other thrombotic disorders. High values have been reported in dogs secondary to neoplasia, inflammatory disease and hemorrhagic effusions, e.g. hemoperitoneum (although most dogs with hemorrhagic effusions have concurrent disease processes that could independently initiate DIC, such as hemangiosarcoma) and dogs with inflammation and neoplasia can also trigger overt DIC. D-dimer just indicates fibrinolysis, regardless of whether this is physiologic (e.g. wound healing) or pathologic (associated with disease) and is not specific for DIC. D-dimer has been evaluated in the cerebrospinal fluid (CSF) as a marker of prior hemorrhage into the central nervous system. One abstract showed that D-dimer values were increased in the CSF of dogs with evidence of hemorrhage, however values were still below the limit of detection of the latex agglutination assays (< 250 ng/mL), requiring the use of more sensitive techniques (which has not been done yet). As far as physiological increases in D-dimer goes, D-dimer concentrations have been examined in dogs immediately and 24 hours after surgery (spay/castration or after elective or traumatic orthopedic procedures). Although there were only 15 dogs in each group, D-dimer concentrations were not consistently increased in each group, 24 hours after surgery. However, results in individual dogs could not be tracked and it is possible that the average results for each group masked individual changes. Increases were seen in the neuter group, immediately after surgery (Shipov et al 2018).
- Cats: D-dimer has been evaluated in cats with various diseases, including sepsis and cardiac disease, which are conditions associated withpathologic thrombosis or thromboembolism. D-dimer concentrations are higher in septic versus non-septic cats, but only 18% of septic cats had classic laboratory criteria of DIC (Klainbart et al 2017). although these criteria may be inadequate for DIC diagnosis in cats). In cardiac disease, D-dimer concentrations were not higher in cats with asymptomatic hypertrophic cardiomyopathy versus healthy cats (Bédard et al 2007) and D-dimer concentrations were not consistently increased in cats with detectable thrombi or acute aortic thromboembolism (Stokol et al 2008). We have seen high D-dimer levels in cats with conditions associated with DIC, e.g. feline infectious peritonitis, suggesting that D-dimer may still be useful to detect thrombosis in some feline diseases. In a study of sick cats with and without classic criteria of DIC, D-dimer concentrations were increased in 75% and 44% of cats with and without DIC, respectively. Concentrations were also increased in 33% of healthy cats (Tholen et al 2009). Thus, the utility of D-dimer concentration for detection of thrombosis or diagnosis of DIC is unclear in cats.
- Horses: D-dimer concentrations are increased in plasma in horses with severe colic, particularly due to inflammatory or strangulating conditions (Monreal et al 2000, Cesarini et al 2010, Cesarini et al 2014) and is a sensitive diagnostic test for the presence of underlying DIC (Stokol et al 2005). In some studies, a high D-dimer was a negative prognostic indicator for outcome in these horses (Monreal et al 2000, Cesarini et al 2010, Nikvand et al 2019). D-dimer concentrations can also be used as markers of hypercoagulability, such as in horses with atrial fibrillation (de Solís et al 2016) and equine herpesvirus type-1 infection (Goehring et al 2013). D-dimer concentrations are increased in extravascular spaces, supporting extravascular fibrinolysis, such as in joint fluids with sepsis and peritoneal fluid with colic (Delgado et al 2007, Ribera et al 2011, Andreassen et al 2017). D-dimer concentrations also increase post-operatively after surgical treatment of colic (Feige et al 2003).
Viscoelastic testing
As a natural part of viscoelastic testing, the clot that forms within the rotational cup will begin to lyse, given sufficient time. The analyzers provide values for percentage lysis at 30 (Ly30) and 60 (Ly60) minutes. There are differences in the sensitivity of methods (thromboelastometry with the ROTEM or thromboelastography with the TEG) in sensitivity to fibrinolysis, with the latter being less sensitive. Clot lysis is also affected by the trigger for clot formation in these assays. For example, with the TEG the average % lysis at 30 and 60 minutes was <7% and <15% in 20 control dogs with tissue factor, contact (native blood) or kaolin as activators, i.e. <7% or <15% of the clot has lysed naturally by 30 and 60 minutes, respectively. The assays, however, had high analytical variation (ranging from 75% to a massive 755% with native blood activated by contact) (Spodsberg et al 2013). The high variation limits the usefulness of the TEG for evaluation of fibrinolysis, unless tPA is added (see below). In dogs, the maximum % clot lysis for thromboelastometry with the ROTEM ranges from 0-14% and 0-3% with tissue factor and ellagic acid (as an activator of the intrinsic pathway; analogous to kaolin in the TEG) as activators (Sigrist et al 2018). In 23 cats, the maximum clot lysis at 60 minutes was 0-14% and 0-10% with tissue factor and ellagic acid. However, clot lysis in cats may be affected by clot retraction which mimics fibrinolysis (Marly-Voquer et al 2017). In one study in which ROTEM was used in 8 cats (likely due to bleeding symptoms), fibrinolysis was higher than the latter established intervals with tissue factor activation in all 8 cats (many had hemorrhage from trauma, hepatic amyloidosis, cancer, post-ovariohysterectomy and snake envenomation). However, addition of aprotonin, a fibrinolytic inhibitor, resulted in more clot lysis in 2 of 4 cats in which it was done (50%), suggesting that, in many cats, this was due to clot retraction (the authors attributed these findings to an insufficient dose of aprotonin). In contrast, excess fibrinolysis was not seen in these 8 cats with ellagic acid as an activator. In the same study, similar findings were seen in 36 dogs (many of which had bleeding symptoms from Angiostrongylus vasorum, cancer and liver disease) in which ROTEM was performed (likely due to suspicion of a bleeding disorder), in that addition of aprotonin resulted in more clot lysis in some dogs and only 30% of dogs had more fibrinolysis than established intervals (from 48 dogs) with ellagic acid. In addition, animals with higher % lysis had hypocoagulable profiles and less firm clots formed. Although this was attributed to excessive fibrinolysis, the excessive fibrinolysis may actually be a consequence of hypocoagubility and not the cause of less firm clots as speculated. Thus, it is difficult to confirm these animals had excess fibrinolysis at all (Sigrist et al 2018).
tPA-induced fibrinolysis
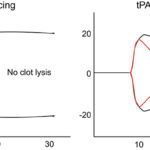
The addition of tissue plasminogen activator (tPA) to clotted plasma triggers fibrinolysis, the rate and extent of which can be measured spectrophotometrically (changes in turbidity) or with viscoelastic-based clotting methods (e.g. thromboelastography [TEG] or thromboelastometry) (Dengate et al 2013, Spodsberg et al 2013, Fletcher et al 2014, Jeffery et al 2017, Sigrist et al 2017). The concentration of fibrinogen and thrombin in the sample alters the structure and strength of the fibrin clot and will affect the rate of fibrinolysis, although one study showed a similar rate of lysis with increasing fibrinogen concentrations (up to 800 mg/dL – higher concentrations can be seen in sick animals, with unknown effects on fibrinolysis [Jeffery et al 2017]). The concentration of tPA is also important as higher concentrations inhibit clot formation (Spodsberg et al 2013, Fletcher et al 2014). For these samples, clotting must first be initiated. This can be done with addition of calcium in native whole blood (i.e. relying to some extent on contact activation from the plastic surfaces of the machine, addition of calcium and tissue factor or a contact activator, such as kaolin, to activate extrinsic and intrinsic pathways, respectively, using 10 pg/mL tPA (Spodsberg et al 2013). In the latter study, % lysis at 30 or 60 minutes was evaluated in healthy dogs and 20 dogs with diseases known to predispose to thrombosis (e.g. inflammation, protein-losing conditions, diabetes mellitus, hyperadrenocorticism or cancer). With the addition of tPA (10 pg/uL final concentration), the % lysis averaged between 36 and 50% at 30 minutes (range, 3-60%) and 73-83% (range, 43-94%) at 60 minutes with tissue factor, kaolin or contact (native blood with only calcium) in the 20 control dogs. The analytical variation was far less (and more acceptable to some degree) with the addition of tPA and kaolin or tissue factor activation (7-14% with kaolin activation, 12-27% with tissue factor activation). Analytical variation was still unacceptably high for native blood (43-115%), indicating an activator should be added. The % lysis at 30 minutes was lower in diseased dogs (suggesting resistance to fibrinolysis, which would predispose to thrombosis) although results overlapped with healthy dogs, regardless of the method of clot initiation, and the study did not indicate if there were disease-associated differences in this “resistance to fibrinolysis”. The study also showed that the analytical variation was high in the healthy dogs (14% and 27% for kaolin- and tissue factor-triggered clotting, respectively, and 115% with no initiator for % lysis at 30 minutes). Resistance to fibrinolysis could be explained by formation of thicker fibrin fibers, such as those formed from higher fibrinogen or thrombin concentrations) versus a loss of fibrinolytic inhibitors. In 28 dogs with spontaneous hemoperitoneum (mostly due to cancer, particularly hemangiosarcoma), tissue factor-induced TEG with 50 U/ml tPA in whole blood showed hypocoagulability (particularly decreased maximum amplitude, which correlated to fibrinogen and platelet concentrations on multiple linear regression) and increased fibrinolysis (with less of the fibrin clot remaining at 30 and 60 minutes) (Fletcher et al 2016). However, the PT and APTT were also prolonged, so the TEG did not add much in this cohort for hypocoagulability, and D-dimer concentrations were high, indicating active fibrinolysis. So routine coagulation tests told the same story. Since most of the dogs in the latter study had hemangiosarcoma, they were likely in DIC, although the authors postulated that the dogs were suffering from acute coagulopathy associated with trauma and shock. It is also possible that the observed hyperfibrinolysis was due to formation of softer thinner clots (from the hypocoagulability) that are more susceptible to fibrinolysis. Aside from whole blood, TEG-based tPA-induced fibrinolysis can be done in citrated plasma and, with this technique, dog plasma is more hypercoagulable and hyperfibrinolytic than human plasma (Fletcher et al 2014). The latter assay has been used to test the in vivo efficacy of the fibrinolytic inhibitor, ε-aminocaproic acid, in dogs (Brown et al 2016).
With optical-or turbidometric-based assays, either a combination of tissue factor, phospholipid, and calcium (Jeffery et al 2017) or thrombin in a calcium buffer (Dengate et al 2013) can be added to plasma to induce clotting, with application of exogenous tPA to induce fibrinolysis. With the latter assay, thrombin-induced coagulation can be assessed (called “overall coagulation potential”) by excluding tPA. The optical-based methods can measure the delay until fibrin formation (less delay in canine than human plasma), the rate of fibrin formation (change in optical density over time or the slope or based on the time taken to reach 50% of maximum absorbance) and maximum fibrin formation (maximum absorbance, less in canine than human plasma), as well as the rate of decrease in absorbance after tPA (e.g. based on a decrease in 50% from maximal absorbance) and “overall hemostasis potential” (area under the fibrin formation and lysis curve). The maximum absorbance and slope were correlated to fibrinogen concentration and results were affected by moderate to marked hemolysis (negative effect), lipemia and bilirubin (negative interference at 15 mg/dL) (Dengate et al 2013, Jeffery et al 2017). Mild hemolysis (90 mg/dL hemoglobin) or icterus (1.9 mg/dL bilirubin) did not affect results more than expected for analytical variation (Jeffery et al 2017). For the delay, the analytical variation was high (up to 42%), however the analytical variation of the other results was <16% (Dengate et al 2013, Jeffery et al 2017). Addition of exogenous heparin and ε-aminocaproic acid showed expected results (inhibition of clotting for the former and inhibition of fibrinolysis for the latter) (Jeffery et al 2017).
Related links
- Further information is available on the D-dimer assay offered by the Animal Health Diagnostic Center’s Comparative Coagulation Laboratory of Cornell University.