Bicarbonate is the major extracellular buffer in the body. It is present in all body fluids and can be generated from CO2 and H2O in the presence of carbonic anhydrase. Bicarbonate on the chemistry panel gives an indication of acid-base status, but does not replace blood gas measurement as it does not supply information about the respiratory component of acid-base status or the pH of the animal. Bicarbonate values on a chemistry panel should always be interpreted with the anion gap, which is a calculated result, and the corrected chloride. The anion gap and corrected chloride provides useful information for delineating causes of metabolic acidosis (loss or titration of bicarbonate) and can give you an indication of a mixed acid-base disturbance. Note, that this page refers to bicarbonate measurement with the chemistry analyzer and not a blood gas analyzer. For the latter, refer to laboratory detection page.
Method of measurement
The following method is used at Cornell University to measure bicarbonate on our automated chemistry analyzer.
Bicarbonate can also be measured on the blood gas analyzer, which uses ion selective electrodes to measure pH and hydrogen (and then calculates the bicarbonate from pH and hydrogen concentration).
Reaction type
Blanked end-point reaction
Procedure
This is a two step reaction that starts with phosphoenolpyruvate carboxylase (PEPC) catalyzing the oxidation of phosphoenolpyruvate (PEP) to oxaloacetate, in the presence of HCO3–. This first reaction is coupled to a second reaction that involves the transfer of a H+ from an nicotinamide adenine dinucleotide (NADH) analog to oxaloacetate using malate dehydrogenase (MDH). The lowered levels of NADH analog causes an absorbance decrease at 415 nm, which directly correlates to the concentration of HCO3– in the sample.
The reactions are illustrated below:
PEP + HCO3 PEPC > Oxaloacetate + H2PO3
Oxaloacetate + NADH analog + H+ MDH > Malate + NAD+ analog
Units of measurement
The concentration of bicarbonate is is expressed in mEq/L (conventional units) and mmol/L (SI units). The conversion formula is essentially one to one or as shown below:
mEq/L x 1 = mmol/L
Sample considerations
Sample type for measurement on a chemistry panel
Serum and plasma.
Sample type for measurement on a blood gas panel
Heparin-anticoagulated blood (see laboratory detection page)
Anticoagulant
Heparin (lithium is usually used, but sodium or ammonium heparin can also be used)
Stability
Bicarbonate levels in human samples are stable for several days at 2 – 8 °C, when separated from erythrocytes and stored tightly stoppered. It has been shown that bicarbonate levels in serum stored at -20 °C or -80 °C are stable for up to 6 months.
Interferences
- Lipemia: Severe lipemia (≥500 lipemia index) may falsely decrease bicarbonate concentrations.
- Hemolysis: Moderate hemolysis may decrease bicarbonate concentrations (≥200 hemolysis index)
- Icterus: May possibly decrease bicarbonate concentrations (icteric index: >60 for conjugated and >50 for unconjugated bilirubin)
Test interpretation
Increased bicarbonate concentration
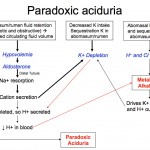
Increased levels of bicarbonate usually indicates a metabolic alkalosis and is usually secondary to chloride depletion, i.e. will be associated with a corrected hypochloremia (chloride disproportionately lower than sodium).
- Artifact: Artifactual causes for an increased HCO3− are quite rare but we occasionally see this in mailed-in samples from large animals (ruminants, particularly). The reason for these changes is unexplained. Very high concentrations of pyruvate and lactate dehydrogenase (both of which can contribute to the enzymatic reaction used above for bicarbonate measurement) secondary to severe muscle injury may falsely increase bicarbonate concentrations (Collins et al 1998) and decrease the anion gap, but this is very rare.
- Iatrogenic: Administration of bicarbonate-containing solutions.
- Pathophysiologic: This usually indicates a metabolic alkalosis. This can be primary condition or secondary to a primary respiratory acidosis.
- Primary chloride-depleted metabolic alkalosis: This condition develops when HCl-rich gastric or abomasal secretions are lost in vomiting or are sequestered. This is the most common metabolic acid-base disturbance in adult dairy cattle and is infrequent in horses. It is commonly seen in dogs and cats with causes of vomiting (of gastric contents) and is often concurrently observed with a primary titration high anion gap acidosis from L-lactate production due to concurrent hypovolemia or the cause of the vomiting (e.g. uremic acidosis), i.e. there is a mixed disturbance. With a primary metabolic alkalosis, both H+ and Cl− are lost (loss of an acid = alkalosis). Gastric cells continue to produce HCl, a reaction that generates sodium bicarbonate in plasma (for more on the exact mechanism, refer to types of acid-base disturbances), resulting in a metabolic alkalosis. The net effect of acid (H+) loss and base gain (HCO3–) is an increase in blood pH, which may result in alkalemia (if pH increases above the reference interval for that species). The hallmarks of metabolic alkalosis are a corrected hypochloremia and increased bicarbonate concentration (with the changes being roughly proportional), unless there is a complicating concurrent metabolic acidosis (which frequently happens with disorders causing gastrointestinal HCl loss), then bicarbonate concentration may be normal. So the best laboratory indicator of a metabolic alkalosis is a low corrected chloride. In a metabolic alkalosis, the kidney should excrete the excess bicarbonate resulting in a high urine pH. However due to the hypochloremia, there is increased sodium delivery to the collecting tubules, the net effect of which is acid excretion (with bicarbonate retention). Both reduced NaCl (reduced salt sensing by macula densa) and hypovolemia (from fluid losses associated with the cause of vomiting or sequestration) will also stimulate aldosterone secretion, which will further promote acid loss from the kidney. Hypokalemia exacerbates these effects (see image above). The renal response to a chloride-depleted metabolic alkalosis is actually to excrete acid, resulting in a so-called paradoxic aciduria, because this is the last thing the kidney should be doing. So to help the kidney do its job to excrete excess bicarbonate in a chloride-depleted alkalosis, give the animal chloride (with fluids) and supplemental potassium (since the latteris also low due to the primary disease).
- Causes of a primary chloride-depleted metabolic alkalosis are the following:
- Gastrointestinal losses or “sequestration” of chloride or hydrochloric acid:
- Vomiting: In small animals, vomiting due to pyloric obstruction, e.g. foreign body or some cases of gastric dilatation-volvulus, causes metabolic alkalosis. In contrast to gastric secretions, duodenal and pancreatic secretions are very rich in HCO3–. Therefore, if small intestinal contents as well as gastric secretions are lost in vomiting (which is more often the case), then metabolic acidosis, rather than alkalosis, develops.
- Gastric reflux in horses: This would lose hydrochloric-rich gastric secretions and is due to various causes. Ileus can also induce the same abnormalities. Metabolic alkalosis is uncommon in horses.
- Gastric obstruction, torsion or rupture: This can occur in dogs (e.g. gastric dilatation volvulus) and horses (e.g. secondary to proximal duodenal obstruction, enteritis or bloat).
- Abomasal atony, torsion or displacement, or “reflux”: Metabolic alkalosis is the most frequent acid-base abnormality in adult cattle and is usually due to either a displaced abomasum or abomasal atony (which can occur with vagus indigestion or in sick cattle with other disorders that do not primarily affect the gastrointestinal tract via unknown mechanisms). Any other disorder, in which abomasal outflow is impaired or obstructed. e.g. abomasal adhesions, abomasal inflammation, pressure on the abomasum from a fetus in advanced pregnancy or proximal duodenal obstruction (causes a severe metabolic alkalosis due to continued secretion of hydrochloric acid), can result in sequestration of HCl and metabolic alkalosis.
- Sweat loss: In horses, hypochloremia, hypokalemia, and metabolic alkalosis can develop when overexerted, through loss of water and chloride-rich electrolytes (KCl) in sweating.
- Renal loss: Loop diuretics can result in loss of excess chloride in relation to sodium (via inhibiting the Na-K-2Cl carrier, i.e. two chlorides are lost for one sodium) and a metabolic alkalosis may result because of increased sodium delivery to the collecting tubules (with resultant excretion of acid and retention of bicarbonate). This may also occur with thiazide diuretics, which block the NaCl carrier in the early distal nephron.
- Gastrointestinal losses or “sequestration” of chloride or hydrochloric acid:
- Causes of a primary chloride-depleted metabolic alkalosis are the following:
- Primary non-chloride-dependent metabolic alkalosis: This is a rare condition caused by excess aldosterone (which stimulates acid excretion in the collecting tubules). The alkalosis is generally mild unless there is concurrent hypochloremia.
- Secondary or compensatory metabolic alkalosis: This is in compensation for a primary respiratory acidosis. In this case, the kidney excretes ammonium chloride (by generating ammonia from glutamine in the proximal tubules which combines with filtered chloride, which is then excreted, and by increasing hydrogen excretion in the distal tubules, see image below). New bicarbonate is generated in blood, resulting in a secondary or compensatory metabolic alkalosis. This offsets the acidemia that results from increased carbon dioxide from a primary respiratory acidosis. The kidney is so good at excreting ammonium chloride and promoting distal tubular acid excretion that, in the dog at any rate, it can actually correct the pH back to normal (given sufficient time, around 30 days) in a chronic respiratory acidosis (usually compensation does not return pH to normal).
- Primary chloride-depleted metabolic alkalosis: This condition develops when HCl-rich gastric or abomasal secretions are lost in vomiting or are sequestered. This is the most common metabolic acid-base disturbance in adult dairy cattle and is infrequent in horses. It is commonly seen in dogs and cats with causes of vomiting (of gastric contents) and is often concurrently observed with a primary titration high anion gap acidosis from L-lactate production due to concurrent hypovolemia or the cause of the vomiting (e.g. uremic acidosis), i.e. there is a mixed disturbance. With a primary metabolic alkalosis, both H+ and Cl− are lost (loss of an acid = alkalosis). Gastric cells continue to produce HCl, a reaction that generates sodium bicarbonate in plasma (for more on the exact mechanism, refer to types of acid-base disturbances), resulting in a metabolic alkalosis. The net effect of acid (H+) loss and base gain (HCO3–) is an increase in blood pH, which may result in alkalemia (if pH increases above the reference interval for that species). The hallmarks of metabolic alkalosis are a corrected hypochloremia and increased bicarbonate concentration (with the changes being roughly proportional), unless there is a complicating concurrent metabolic acidosis (which frequently happens with disorders causing gastrointestinal HCl loss), then bicarbonate concentration may be normal. So the best laboratory indicator of a metabolic alkalosis is a low corrected chloride. In a metabolic alkalosis, the kidney should excrete the excess bicarbonate resulting in a high urine pH. However due to the hypochloremia, there is increased sodium delivery to the collecting tubules, the net effect of which is acid excretion (with bicarbonate retention). Both reduced NaCl (reduced salt sensing by macula densa) and hypovolemia (from fluid losses associated with the cause of vomiting or sequestration) will also stimulate aldosterone secretion, which will further promote acid loss from the kidney. Hypokalemia exacerbates these effects (see image above). The renal response to a chloride-depleted metabolic alkalosis is actually to excrete acid, resulting in a so-called paradoxic aciduria, because this is the last thing the kidney should be doing. So to help the kidney do its job to excrete excess bicarbonate in a chloride-depleted alkalosis, give the animal chloride (with fluids) and supplemental potassium (since the latteris also low due to the primary disease).
Decreased bicarbonate concentration
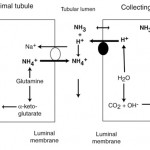
Unless there is clear evidence of sample collection or storage issues or a respiratory disorder that would cause accumulation of CO2, low bicarbonate is presumptive evidence of metabolic acidosis. Diseases that cause loss or titration of HCO3– must then be considered. Evaluation of the anion gap and corrected chloride concentration helps distinguish between these causes of a metabolic acidosis, although both may be occurring concurrently resulting in a mixed acid-base disorder. Metabolic acidosis is the most common acid-base disorder encountered in veterinary practice.
- Artifact: False decreases in bicarbonate occurs in aged samples. This is usually due to lactate production by cells with anaerobic metabolism, with buffering of bicarbonate in the plasma within the tube. Over-dilution with heparin and prolonged venous stasis will also decrease bicarbonate.
- Iatrogenic: Administration of ammonium chloride will cause a hyperchloremic metabolic acidosis.
- Pathophysiologic: A decreased bicarbonate indicates a metabolic acidosis. This can be a primary disorder from loss or consumption of bicarbonate or a secondary disorder in compensation for a primary respiratory alkalosis. These can be distinguished by measuring the partial pressure of carbon dioxide on a blood gas analysis, evaluating the animal for a respiratory disorder or cause of metabolic acidosis (see below) and examining the anion gap and corrected chloride concentration.
- Titration or consumption of bicarbonate metabolic acidosis: This is also called a high anion gap metabolic acidosis and is always a primary condition (it is never compensatory in response to a primary respiratory alkalosis). Bicarbonate consumption by organic acids that lack chloride as the anion (unmeasured anion) will increase the anion gap (and corrected chloride will be unchanged unless there is another acid-base abnormality or disease condition affecting chloride). A titration or high anion gap acidosis is by far the most common cause of metabolic acidosis in small animals, camelids, horses and adult ruminants (rare in the latter). Acids that are unmeasured anions include lactate, noncarbonic acids (sulfates, phosphates and citrates), ketones (diabetic ketoacidosis), toxins (ethylene glycol and its metabolites, methanol) and drugs (acetylsalicylic acid). In these conditions, bicarbonate is consumed in buffering the accumulated noncarbonic acid, leaving the anion of the acid in its place (thus increasing the anion gap).
- Lactic acidosis: Usually results from decreased tissue perfusion with production of L-lactate (anaerobic glycolysis increases lactate production), e.g. hypovolemia, hypoxia. Lactic acidosis also results from grain overload in cattle.
- Acute kidney injury or chronic renal disease (usually the later stages) resulting in failure may result in a high anion gap metabolic acidosis due to retained noncarbonic acids that are normally filtered by the kidney (e.g. phosphates, citrates, hippurates).
- Ketoacidosis: Ketones (acetoacetic acid, acetone, β-hydroxybutyrate) accumulate in unregulated diabetic patients or camelids or ruminants with negative energy balance (e.g. transition dairy cows, camelids that are stressed, particularly in late pregnancy). The lack of insulin or insulin resistance in diabetes mellitus type I or II, respectively, leads to increased lipolysis of adipose tissue with enhanced presentation of non-esterified fatty acids (NEFA) to the liver. These fatty acids are used for energy, incorporated into lipoproteins (VLDL) or shunted into ketone production. In ruminants and camelids, negative energy balance causes excessive lipolysis, which is compounded by stress (hormones such as corticosteroids and epinephrine stimulate hormone-sensitive lipase which breaks down fat stores). Horses do not usually develop ketosis due to poorly developed ketone pathways.
- Toxicity: Dogs and cats with antifreeze (ethylene glycol) poisoning have a high anion gap metabolic acidosis caused by metabolites of ethylene glycol. They also have a high osmolal gap (measured minus calculated osmolality > 30) because ethylene glycol and its metabolites are unmeasured osmols but are not included in the equation to calculate osmolality (based on sodium, potassium, urea nitrogen and glucose).
- Bicarbonate loss metabolic acidosis: This is also called a hyperchloremic metabolic acidosis because it is associated with a high corrected chloride and a normal anion gap. This can be a primary or secondary disorder.
- Primary bicarbonate loss metabolic acidosis: Bicarbonate can be lost through the gastrointestinal tract or kidneys.
- Gastrointestinal tract: Vomiting of bicarbonate-rich solutions (biliary or pancreatic fluids), sequestration of bicarbonate within the gastrointestinal tract (e.g. ileus in horses), diarrhea (usually secretory), loss of saliva particularly in cattle (and to a lesser extent horses). Saliva is high in bicarbonate, so loss of saliva through inability to swallow (e.g. rabies, choke) can result in a metabolic acidosis. In these conditions, water and sodium are lost concurrently, hence hypovolemia ensues. As a response to hypovolemia, the kidney absorbs sodium and water. Sodium is absorbed with chloride, which then increases in blood, resulting in hyperchloremia with a normal anion gap.
- Kidneys: Filtered bicarbonate is usually absorbed (reclaimed) in the proximal renal tubules with filtered sodium or acids (e.g. phosphate, called titratable acidity) or ammonia generated by the proximal tubules or passively excreted by the distal tubules (see image above). Defective proximal renal tubule absorption of bicarbonate can cause a hyperchloremic bicarbonate loss acidosis, e.g. proximal renal tubular acidosis (also called Fanconi’s syndrome). Addison’s disease can also result in a hyperchloremic metabolic acidosis because aldosterone promotes hydrogen excretion in the distal tubules of the kidneys (lack of aldosterone would promote hydrogen retention or an acidosis). Distal renal tubular acidosis will also result in a normal anion gap hyperchloremic metabolic acidosis due to defective excretion of hydrogen by distal tubules.
- Secondary bicarbonate loss metabolic acidosis: This occurs when the kidney reduces acid excretion (by decreasing ammonia generation in the proximal convoluted tubules or H+ATPase activity in the collecting tubules, subsequently causing filtered bicarbonate to be excreted and chloride to be retained) because of a primary respiratory alkalosis. The kidney also promotes bicarbonate loss by stimulating the pendrin pump (chloride/bicarbonate exchanger) in the apical membrane of type B intercalated cells. This excretes bicarbonate in the urine in exchange for chloride. The kidney is so effective at excreting bicarbonate when compensating for a primary respiratory alkalosis, that this compensatory response can actually correct the pH (given sufficient time, around 14 days) in dogs. It is unknown if this happens in other species. For more information on how the kidney handles bicarbonate absorption and hydrogen excretion, refer to the renal portion of this website.
- Primary bicarbonate loss metabolic acidosis: Bicarbonate can be lost through the gastrointestinal tract or kidneys.
- Titration or consumption of bicarbonate metabolic acidosis: This is also called a high anion gap metabolic acidosis and is always a primary condition (it is never compensatory in response to a primary respiratory alkalosis). Bicarbonate consumption by organic acids that lack chloride as the anion (unmeasured anion) will increase the anion gap (and corrected chloride will be unchanged unless there is another acid-base abnormality or disease condition affecting chloride). A titration or high anion gap acidosis is by far the most common cause of metabolic acidosis in small animals, camelids, horses and adult ruminants (rare in the latter). Acids that are unmeasured anions include lactate, noncarbonic acids (sulfates, phosphates and citrates), ketones (diabetic ketoacidosis), toxins (ethylene glycol and its metabolites, methanol) and drugs (acetylsalicylic acid). In these conditions, bicarbonate is consumed in buffering the accumulated noncarbonic acid, leaving the anion of the acid in its place (thus increasing the anion gap).