Physiology
In health, approximately 60% of an adult animal’s body weight is water, but this varies with species, age, sex, breed, and body composition. For example, in humans, total body water decreases with age and is lower in women than in men. Neonatal dogs and cats have higher total body water content (about 80%) compared to adults. In greyhounds, approximately 70% of their total body weight is water (likely due to low body fat content). Fat has a lower water content than lean tissue. This is the reason behind estimating fluid needs on “lean body weight” and not total body weight.
Water is the major component of all body fluids, which are distributed into several physically distinct compartments, including the intracellular fluid (ICF) and extracellular fluid (ECF) compartments. The ECF compartment can be split into interstitial fluid and plasma compartments. The fluids in each compartment equilibrate by multiple mechanisms to maintain homeostasis. The largest volume of fluid in the body is inside cells (the intracellular fluid or ICF compartment) and makes up about 2/3 of total body water. Any fluid not inside of cells is in the extracellular fluid (ECF) compartment (the remaining 1/3 of total body water). In most disease states, loss of fluids occurs initially from the ECF, particularly from plasma or the intravascular compartment. The 60:40:20 rule can help you remember the distribution of body water: 60% of body weight is water, 40% of body weight is ICF, and 20% of body weight is ECF. The ECF is further separated into interstitial fluid (space surrounding cells), intravascular compartment (plasma), and transcellular compartment. Dense connective tissue, cartilage, and bone also contain a small amount of ECF. Most ECF is present in interstitial fluid (around 3/4), with the remaining 1/4 of ECF within blood vessels (intravascular compartment) as plasma. The transcellular fluid compartment is small (about 1% of body weight) and consists of fluids produced by specialized cells (e.g. cerebrospinal fluid, GI fluid, bile, glandular and respiratory secretions, and synovial fluid).
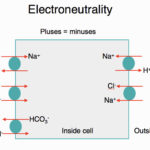
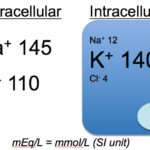
The composition of the ICF and ECF are very different. Solutes are not distributed equally throughout body fluids, due to the vascular endothelium and different permeability of cell membranes for various solutes. Sodium and chloride are much higher in the ECF than potassium, which is higher in the ICF (remember, that in most cells, Na/K transporters pump out and K in). Despite these differences, the total number of all cations and anions in body fluids are equal to maintain electroneutrality.
Osmolality
Osmolality refers to the number of osmotically active particles (osmoles) per kilogram of solvent. In clinical veterinary medicine, osmolality is expressed as milli-osmoles per kg. Osmolarity is simply the concentration per liter of solvent. In biologic fluids, there is a negligible difference between osmolality and osmolarity and the terms are often used interchangeably. Osmolality can be assessed in two ways:
- Measured directly with an osmometer using freezing point (technique used in the clin path lab) or vapor pressure depression.
- Calculated (estimated) if the serum concentrations of Na, K, glucose, and urea nitrogen (UN also referred to as blood urea nitrogen [BUN] or more correctly serum urea nitrogen [SUN] or plasma urea nitrogen [PUN]) are known:
Osmolality = 2(Na + K) + (glucose/18) + (UN/2.8)
with 18 and 2.8 being factors, which convert mg/dL (conventional units) to mmol/L (SI units)
The calculated osmolality is typically about 280-310 mOsm/kg in healthy animals, whereas measured osmolality ranges from 290-310 mOsm/kg in dogs, 290-330 mOsm/kg in cats, and 280-320 mOsm/kg (with minor differences in EDTA- or lithium-heparinized plasma) in horses (Wilkinson 1976).
The osmolal gap is the difference between the measured and calculated serum osmolality:
Osmolal gap = Measured – calculated osmolality
- Normal osmolal gap: < 10 mOsm/kg.
- High osmolal gap: > 10 mOsm/kg. This indicates the presence of unmeasured osmoles (“unmeasured” in terms of the calculation) e.g. ethylene glycol, mannitol or false decreases in sodium or pseudohyponatremia, e.g. marked hyperlipemia or hyperproteinemia.
Effective osmolality or tonicity
Changes in the osmolality of ECF may or may not initiate movement of water between the ICF and ECF. Tonicity refers to the ability of a solution to initiate water movement and is dependent on impermeant or effective osmoles, which do not readily cross most membranes. The most important effective osmoles we deal with are sodium and glucose (glucose is really only an effective osmol in states of insulin deficiency). In contrast, permeant or ineffective osmoles that move across membranes (e.g. urea) do not cause movement of water. Thus, tonicity can be thought of as effective osmolality, which is not necessarily equal to the osmolality. This is because osmolality includes both effective and ineffective osmoles, whereas tonicity only includes effective osmoles. Tonicity can be estimated from the osmolality by subtracting the most abundant ineffective osmole (urea) from the calculated osmolality equation:
Osmolality = 2(Na + K) + (glucose/18)
A solution is termed hypertonic, isotonic, or hypotonic based on higher, equal, or lower concentrations of impermeant solutes compared to normal plasma. For the purposes of this lecture, consider a fluid conceptually as hypotonic, isotonic and hypertonic as follows:
- Hypotonic: Na concentration is lower than plasma (Na content in fluid < water content of fluid), i.e water is lost or gained in excess of sodium.
- Isotonic: Na concentration is similar to plasma (Na content in fluid = water content of fluid), i.e. water and sodium are lost or gained equally.
- Hypertonic: Na concentration is higher than plasma (Na content in fluid > water content of fluid), i.e. sodium is lost or gained in excess of water.
The body senses changes in tonicity (around 1-2%) via osmoreceptors.
ECF volume regulation
The body senses and responds to changes in ECF volume, which is largely governed by sodium concentration, the major extracellular cation. So when you think of sodium, think of water; the two go together. In fact the primary goal of the body with respect to fluids is to maintain effective circulating volume (that within blood vessels or the intravascular compartment). The effective circulating volume is defined as the fluid perfusing tissues and it is the main stimulus for ECF volume regulation, controlling sodium concentrations and water input and output. The effective circulating volume is sensed by low-pressure baroreceptors. There are various types of baroreceptors or pressure receptors:
- High-pressure baroreceptors in the aortic arch and carotid sinus. These respond to high blood pressure by slowing heart rate and inducing vasodilation.
- Low-pressure baroreceptors in the carotid sinus and cardiac atria. These respond to drops in pressure, which are usually secondary to changes in blood volume (this is why some people refer to them as volume receptors, but they are pressure receptors and not volume receptors per se). The low-pressure receptors respond to changes in effective circulating volume of around 5-10%. In conditions of hypervolemia, increased blood volume will stimulate low-pressure receptors in the right cardiac atrium leading to inhibition of ADH secretion and vasodilation.
- Juxtaglomerular apparatus of the kidney: A baroreceptor that senses changes in perfusion pressure in the afferent arteriole of the glomerulus.
The kidneys are the main organ responsible for regulating total body sodium, and do this by resorbing filtered sodium or excreting sodium. The main stimulus for sodium resorption or excretion are changes in volume (hypovolemia, hypervolemia).
The body responds to changes in tonicity and volume status by modulating water intake or excretion (in response to volume and tonicity changes, sensed by baroreceptors and osmo-oreceptors, respectively) and altering sodium intake or excretion (in response to volume changes, sensed by baroreceptors)
- ADH: This is produced in paraventricular and supraoptic neurones in the hypothalamus and moves down the neuron within secretory vacuoles to the posterior pituitary from where it is released. The main drive for ADH release is hypertonicity (sensed by osmoreceptors), but hypovolemia also stimulates ADH release via stimulation of low- pressure baroreceptors in the carotid sinus and atria. ADH acts on principle cells in the collecting tubules of the kidney by binding to a receptor (vasopressin-2, particularly or VP-2) on the basolateral surface. This increases cAMP levels within the cell and stimulates movement of aquaporin-2 (water channel) from the subapical regions within the cytosol to the luminal membrane. Insertion of aquaporin-2 into the luminal membrane allows water to move passively into the cell, where it flows through aquaporins-3 and -4 into the medulla of the kidney due to the high medullary interstitial tonicity. Aquaporin-2 is endocytosed and not always present on the luminal membrane (Boone and Dean 2008). ADH secretion is inhibited by hypertonicity and hypervolemia.
- Thirst reflex: This is stimulated by hypertonicity (osmoreceptors) and hypovolemia (baroreceptors activating angiotensin II) and inhibited by hypotonicity and hypervolemia.
- Renin-angiotensin II-aldosterone system: This is stimulated by baroreceptors in the juxtaglomerular apparatus, responding to hypovolemia, and decreased sodium and chloride absorption in the macula densa, a group of specialized renal tubular epithelial cells located at the junction of the ascending limb of the loop of Henle and the first part of the distal tubule (these sense salt via absorption of NaCl). Activation will promote sodium absorption in the kidney (angiotensin II in proximal tubules and in exchange for potassium in aldosterone-sensitive parts of the distal nephron) and the thirst reflex (angiotensin II)
- Atrial natriuretic peptide: This is stimulated by pressure receptors in the atria in response to hypervolemia and causes vasodilation. It also stimulates sodium and water excretion.
Hypovolemia
This is defined as decreased effective circulating volume and is usually due to fluid losses from the body. The main sources of fluid losses are the gastrointestinal tract (e.g. vomiting, diarrhea) and kidney (e.g. osmotic diuresis), with the skin, third space “losses” or sequestration and respiratory losses being less frequent sources of fluid loss.
The body’s response to hypovolemia is to:
- Retain or resorb sodium in the kidney (then water follows!): The kidney becomes sodium avid!
- Retain water in the kidneys (ADH, Na absorption) or gain water by drinking. Drinking is the main mechanism by which the body gains water.
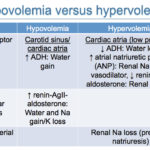
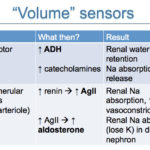
In order to respond, the body must first sense there is a problem, i.e. hypovolemia. This is accomplished baroreceptors in the carotid sinus, left cardiac atria and in the afferent arteriole within the juxtaglomerular complex of the kidneys. These sensors usually kick in when there is an 5-10% reduction in effective circulating volume and cause the following changes:
- Carotid sinus (primary) and left cardiac atria baroreceptors: These sense decreased blood pressure and do the following:
- Stimulate release of antidiuretic hormone (ADH) from the posterior pituitary gland via activation of the vagus nerve. ADH then acts on the collecting tubules of the distal nephron and opens water channels (aquaporin-2) in the luminal membrane. Water moves passively along a concentration gradient into the hypertonic renal medulla, producing concentrated urine and decreasing urine output (you need a hypertonic medulla and functioning tubules for ADH to work properly). The amount of water absorbed is dependent on that filtered, so a decreased GFR will result in less water being filtered to be able to be resorbed under the influence of ADH.
- Increased ADH = water retention (without sodium) in kidneys.
- Stimulate the sympathetic nervous system to release catecholamines, which stimulate proximal tubular sodium and water absorption (α1) and renin release (β1) (see below). They are also vasoconstrictive (which will act to increase blood pressure). Catecholamines are the least important hormone response to hypovolemia.
- Increased catecholamines = sodium and water retention in kidneys.
- Increased catecholamines = stimulation of the renin-angiotension-aldosterone system
- Stimulate release of antidiuretic hormone (ADH) from the posterior pituitary gland via activation of the vagus nerve. ADH then acts on the collecting tubules of the distal nephron and opens water channels (aquaporin-2) in the luminal membrane. Water moves passively along a concentration gradient into the hypertonic renal medulla, producing concentrated urine and decreasing urine output (you need a hypertonic medulla and functioning tubules for ADH to work properly). The amount of water absorbed is dependent on that filtered, so a decreased GFR will result in less water being filtered to be able to be resorbed under the influence of ADH.
- Juxtaglomerular apparatus: Baroreceptors in the afferent arteriole sense decreased arterial perfusion pressure and release renin. Renin stimulates the production of angiotensin II.
- Angiotensin II promotes sodium and water retention by stimulating sodium reabsorption in the proximal tubule and promotes water retention (typically without sodium) by stimulating thirst. Angiotensin II causes vasoconstriction. Angiotensin II also stimulates aldosterone secretion from the zona glomerulosa of the adrenal cortex.
- Increased AgII = sodium and water retention in proximal tubules of the kidney
- Increased AgII = water retention (without sodium) by stimulating thirst
- Increased AgII = aldosterone secretion from adrenal cortex
- Aldosterone acts on the connecting tubules and collecting ducts to increase sodium reabsorption (water will follow) while causing potassium (or hydrogen in states of hypokalemia) excretion into urine. This results in a net gain of sodium and loss of potassium. Aldosterone also directly stimulates H+ excretion (stimulates an H+-ATPase in the distal nephron).
- Increased aldosterone = sodium and water retention
- Increased aldosterone = K+ excretion (H+ will exchange with Na+ in states of hypokalemia)
- Angiotensin II promotes sodium and water retention by stimulating sodium reabsorption in the proximal tubule and promotes water retention (typically without sodium) by stimulating thirst. Angiotensin II causes vasoconstriction. Angiotensin II also stimulates aldosterone secretion from the zona glomerulosa of the adrenal cortex.
Hypervolemia
This is defined as increased effective circulating volume and is usually due to fluid retention. The main sources of fluid retention are the gastrointestinal tract (e.g. drinking) and kidney (e.g. under the influence of ADH).
The body’s response to hypervolemia is to:
- Excrete water in the kidneys or decrease water intake (inhibit thirst)
- Excrete sodium in the kidney (then water follows sodium out!)
In order to respond, the body must first sense there is a problem, i.e. hypervolemia. This is accomplished by low-pressure baroreceptors in the cardiac atrial sinus and increased renal arterial pressure, which cause the following changes:
- Cardiac atrial baroreceptors (mostly in the right atrium): These sense atrial distention and do the following:
- Inhibit ADH.
- Decreased ADH = Water excretion (without sodium) in kidney.
- Stimulate release of atrial natriuretic peptide (ANP) from atrial myocytes. ANP inhibits sodium resorption in the collecting ducts and the secretion of renin and AgII and inhibits the renal responses to AgII and ADH. It is also a vasodilator (the latter may be its main mechanism of action).
- Increased ANP = Sodium and water excretion via decreasing renin, AgII, sodium and water absorption.
- Inhibit ADH.
- Juxtoglomerular apparatus: These sense increased renal arterial pressure and results in increased sodium and water excretion by the kidney. This is called pressure natriuresis and is independent of any hormonal stimulation. This is likely the main mechanism responsible for excretion of sodium and water by the kidney in states of hypervolemia.
Osmolality regulation
The body is able to sense and respond to changes in plasma osmolality (equivalent to tonicity), which is largely determined by serum sodium concentration (being the most abundant extracellular cation and effective osmol). Note that glucose also acts as an effective osmol in pathologic conditions, where it cannot be taken up into cells (e.g. lack of insulin as in diabetes mellitus or insulin resistance). The body responds to changes in tonicity by water excretion or gain and not by altering sodium balance (in contrast to changes in ECF volume, where changes in sodium and water balance occurs). As for ECF volume changes, the main organ responsible for control of osmolality is the kidney. Changes in effective osmolality or tonicity are sensed by osmoreceptors in the hypothalamus, which shrink or swell with hyperosmolality or hypo-osmolality, respectively. Osmoreceptors respond to a change in plasma osmolality as small as 1-2% (1-3% in dogs). Activation of osmoreceptors causes changes in the thirst response and ADH release and actually stimulate maximal ADH release, demonstrating how tightly osmolality is regulated. Conversely shrinkage of osmoreceptors or hypo-osmolality inhibits the thirst response and ADH release.
Hypotonicity (effective hypo-osmolality)
This is defined as decreased amounts of effective osmols, primarily sodium. Sodium can be low because of:
- Dilution by water or water gain (water > sodium):
- Increased water intake, e.g. primary polydipsia, hypotonic fluid administration. Patients are typically normovolemic.
- Renal retention of water, e.g. syndrome of inappropriate ADH release (patients are typically normovolemic) or perceived volume depletion (e.g. cirrhosis, nephrotic syndrome, congestive heart failure-patients are typically hypervolemic).
- Excessive electrolyte loss: Patients are typically hypovolemic. When of sufficient degree (5-10%), hypovolemia will drive the body’s responses.
- Hypertonic losses (sodium in excess of water in lost fluid): Gastrointestinal system, specifically secretory diarrhea or sequestration (ileus, displaced abomasa in cattle), or cutaneous losses, such as extensive burns, excessive sweating in horses.
- Hypotonic losses (water in excess of sodium in lost fluid): This is the most common type of fluid loss and occurs in the gastrointestinal tract, kidney, body cavities (“sequestration in third space”), respiratory system, and skin. To result in hyponatremia, this is accompanied by a thirst (in particular) or ADH response to retain water because there is concurrent hypovolemia sufficient to stimulate sodium and water retentive responses.
- Isotonic fluid losses (water is equal to sodium): This should not impact electrolyte concentrations unless hypovolemia ensues, whereby the body’s response (ADH, thirst) causes water intake in excess of sodium which may result in hyponatremia.
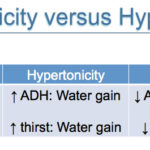
The body’s response to hypo-osmolality is:
- Inhibition of ADH release: Decrease water retention (which will increase sodium and osmolality).
- Inhibition of the thirst response: Decrease water intake (which will increase sodium and osmolality).
However, if a sufficient degree of hypovolemia or decreased effective circulating volume is concurrent, these dominate and ADH and aldosterone are released and the thirst response is stimulated, which tends to worsen the hyponatremia.
Hypertonicity (effective hyperosmolality)
This is defined as increased amounts of effective osmols, primarily sodium, with glucose being an effective osmol with lack of or inhibition of insulin. Sodium can be high because of:
- Excess salt intake (sodium > water), e.g. salt poisoning in ruminants, administration of hypertonic saline.
- Decreased water intake: Lack of access to water or unable to drink, e.g. neurologic disorder, frozen water bowl.
- Increased “pure” water loss (water > sodium): Loss of water through the kidneys, such as by lack of ADH (central diabetes insipidus) or a defective renal tubular response to ADH (e.g. medullary solute washout), also called renal diabetes insipidus.
- Hypotonic fluid losses (water > sodium): This is the most common type of fluid loss and occurs via the gastrointestinal tract (e.g. vomiting, diarrhea), kidney (e.g. osmotic or chemical diuresis), sequestration (“third space”), respiratory system, and skin. Hypotonic fluid losses result in transient increases in sodium concentration. Because fluid losses cause concurrent hypovolemia, when of sufficient magnitude, the corrective responses for hypovolemia kick in (ADH, catecholamines, renin-angiotensin-aldosterone) and water and sodium resorption occur, causing retention of water and sodium, which decreases the sodium concentration and osmolality (to normal or even “overshoots” to result in hypo-osmolality). Hypertonicity will dictate the body responses in states of hypervolemia. As stated above, hypotonic fluid losses can result in a persistent hypernatremia if there is inadequate water intake, in particular, or, less commonly, an inadequate ADH response.
The body’s response to hypertonicity will lead to water retention by stimulation of:
- ADH release: Retention of water without sodium. ADH release is stimulated when osmolality exceeds >300 mOsm/kg in dogs (334 mOsm/kg in 6 helathy dogs, Heine et al 2004).
- Thirst response: Retention of water without sodium.
This will occur even in states of volume expansion (which normally inhibits osmoreceptors).
Summary of players
The following players are involved in water balance:
Player | Stimulus | Action | Effect | Inhibited by |
ADH | Hyperosmolality (shrinkage of osmoreceptors) Hypovolemia or decreased effective circulating volume (carotid sinus and atria receptors) Nausea, pain, anxiety, certain drugs |
Binds to vasopressin receptors (VP-2) in principal cells in collecting ducts, increasing cAMP and moving aquaporin-2 to luminal membrane Vasoconstriction Urea absorption in collecting duct |
Water retention Increased urine osmolality (concentration) Contributes to hypertonic medulla |
Hypo-osmolality (swelling of osmoreceptors) Hypervolemia (low-pressure baroreceptors in right cardiac atria) |
Thirst | Hyperosmolality Hypovolemia (angiotensin II) |
Drinking | Water retention | Hypo-osmolality Hypervolemia (atrial natriuretic peptide) Neurologic conditions |
Aldosterone | Angiotensin II (see below) Hyperkalemia ACTH |
Sodium absorption in principal cells in aldosterone-sensitive part of nephron (distal collecting tubule, connecting tubule, cortical collecting tubule) and stimulates basolateral Na/K pump Potassium excretion in kidney |
Sodium and water retention | Inhibitors of renin release |
Angiotensin II via renin | Stimulation of renin secretion (decreased NaCl absorption in the macula densa) Hypovolemia (baroreceptors in juxtaglomerular apparatus) Catecholamines (β1) |
Stimulates thirst Aldosterone secretion with sodium and water retention Promotes sodium absorption in proximal convoluted tubules Vasoconstrictive (e.g. efferent arteriole in glomerulus) |
Water retention Sodium retention Increase blood pressure |
Inhibitors of renin release (e.g. increased renal pressure, atrial natriuretic peptide) |
Atrial natriuretic peptide (ANP) | Hypervolemia (too much atrial stretch, sensed by right atrial baroreceptors) | Vasodilator Inhibits sodium absorption in collecting duct |
Sodium and water loss Decrease blood pressure |
Hypovolemia (less atrial stretch) |
Catecholamines | Hypovolemia (baroreceptors) | Stimulate proximal tubular sodium and water absorption (α1) Stimulate renin release (β1) Vasoconstrictive |
Sodium and water retention Increase blood pressure |
Hypervolemia |
References
- Clinical Physiology of Acid-Base and Electrolyte Disorders by Rose BD and Post DW, 5th edition, 2001. McGraw-Hill, New York, NY.
- Fluid, Electrolyte and Acid-Base Disorders in Small Animal Practice by DiBartola SP. Elsevier-Saunders, St Louis, MO.