Synonyms
Serum alkaline phosphatase (SAP, SALP, ALP) or plasma alkaline phosphatase
Physiology
Alkaline phosphatase (ALP, AP) is a non-specific metallo-enzyme which hydrolyzes many types of phosphate esters at an alkaline pH in the presence of zinc and magnesium ions. The main use of ALP is as a sensitive indicator of cholestasis in the dog (it will increase before bilirubin), however it is non-specific because corticosteroids (exogenous or endogenous “stress”) induces production of this enzyme, with subsequent increases in serum or plasma activity. In the cat however, ALP is a specific indicator of liver disease, whereas in large animals, the enzyme is less useful as it is insensitive, cholestatic disorders are infrequent, and reference intervals are quite broad.
Isoenzymes and isoforms of ALP
There are two isoenzymes (products of different genes) and several isoforms (produced from post-translational modification of isoenyzmes) of ALP. The isoenzymes are produced from intestinal and tissue non-specific ALP genes and differ in amino acid sequence. Isoforms differ in catalytic sites and activity, immunogenicity, and electrophoretic mobility. The major isoforms that can be measured in animals are liver-ALP (L-ALP), corticosteroid-ALP (C-ALP; only in dogs), bone-ALP (B-ALP) and intestinal-ALP (I-ALP), but there are others including leukocyte-ALP and placental-ALP.
- Intestinal-ALP gene/isoenzyme: This produces the C-ALP and I-ALP isoforms. C-ALP is unique to dogs and is induced by corticosteroids (endogenous or exogenous). Even though it is a product of the intestinal gene, it is actually produced in hepatocytes not intestinal epithelium.
- Tissue non-specific-ALP gene/isoenzyme: This produces the remaining isoforms of ALP, including L-ALP, B-ALP, placental-ALP and leukocyte -ALP.
Organ specificity
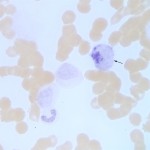
ALP is a cell membrane-bound protein that is present in many tissues. It is anchored to cell membranes by glycophosphatidylinositol (GPI) proteins. Cleavage of these proteins by bile acids, phospholipase D and proteases releases ALP from membranes resulting in increased ALP activity in serum/plasma.
- Liver: Hepatocytes and the epithelium of biliary tract are the source of the L-ALP isoform (all species) and C-ALP isoform in the dog. ALP expression is normally restricted to the canalicular membrane of hepatocytes, but can be induced (secondary to cholestasis typically but also corticosteroids) on the sinusoidal membrane (where it can be readily liberated into blood).
- Bone: This isoform is produced by osteoblasts and will increase in serum in association with osteoblastic activity (young animals, certain bone disorders). High serum concentrations of ALP can also be seen in osteosarcoma, a malignant neoplasm of osteoblasts.
- Intestinal, renal, mammary, placental tissues: These are not usually important sources of increased serum ALP activity, though some placental isoform is present in serum of late pregnant queens (Everett et al 1977) and mares (Dumas and Spano 1980). Mildly increased activity of ALP is seen in dogs with various types of mammary tumors (Karayannopoulou et al 2006).
- Leukocytes: Cell lineage expression is species-dependent. Canine monoblasts (immature monocytes) and eosinophils are rich in ALP, as are horse neutrophils (Stokol et al 2015). The presence of ALP within a cell can be detected by applying an ALP-specific substrate to cytologic smears – this technique (detecting enzymes based on their ability to cleave a substrate) is called cytochemical staining. At Cornell University, we use ALP cytochemical staining as a marker for myeloid cells in acute leukemia. Although neoplastic monoblasts may express ALP in their cytoplasm, high serum or plasma ALP activity is rarely usually seen (Froment and Bédard 2016)). Cytochemical staining for ALP is also used to differentiate osteoblasts from other mesenchymal cells in cytologic aspirates from tumors, i.e. high expression is expected in osteosarcomas, but not chondrosarcomas or fibrosarcomas (Barger et al 2005). We use ALP cytochemical staining to help confirm a diagnosis of osteosarcoma in cytologic specimens.
Half life
The half life of ALP varies depending on the source (isoform) and species (Hoffman and Dorner 1977, Hoffman et al 1977). Isoforms with longer half-lives contribute more to normal and increased levels of serum or plasma ALP, i.e. increased levels of isoforms with very short half life (minutes versus days) are unlikely to be observed on a single blood sample (since the peak may be missed and values will rapidly decreases).
Isoform | Species | Half life (hours) |
Liver (L-ALP) | Canine | 2-3 days (66 hours) |
Feline | 6 hours | |
Steroid (C-ALP) |
Canine | 2-3 days (74 hours) |
Intestinal (I-ALP), kidney, placental | Canine/equine | <6 minutes |
Feline | < 2 minutes | |
Bone (B-ALP) | Canine | 2-3 days |
Other species | ? |
Methods
Below is the method used to measure total ALP activity on our chemistry analyzer at Cornell University.
Reaction type
End-point
Procedure
In a one step reaction, ALP cleaves p-nitrophenyl phosphate into phosphate and p-nitrophenol. The generation of p-nitrophenol is measured photometrically and is proportional to ALP activity. This reaction occurs in the presence of magnesium and zinc ions (thus ALP measurement cannot be performed in EDTA, because this will chelate magnesium and zinc).
p-Nitrophenyl phosphate + H2O ALP > p-nitrophenol (yellow) + phosphate
Units of measurement
Enzyme activity is measured in U/L (U = international unit) and µkat/L (SI units), which is defined as the amount of enzyme that catalyzes the conversion of 1 µmol of substrate per minute under specified conditions. The conversion formula is shown below:
U/L x 0.0167 = µkat/L
Isoform measurement
Differentiation of isoforms can be accomplished by electrophoretic (affinity agarose electrophoresis, immunoassays, cellulose acetate electrophoresis or isoelectric focusing on agarose) or differential inhibition methods using levamisole (inhibits L-ALP and B-ALP especially), heat (inactivates L-ALP and B-ALP) and wheat germ lectin (precipitates B-ALP and C-ALP) (Wellman et al 1982, Syakalima et al 1997, Allen et al 2000, Hatayama et al 2011)
Sample considerations
Sample type
Serum or plasma
Anticoagulant
Heparin (lithium, sodium, ammonium). EDTA cannot be used.
Stability
The stability of ALP activity in human serum and plasma samples is as follows: 7 days at 15 – 25 °C or 2 – 8 °C, and 2 months at frozen at about -20°C. In animal plasma or serum, ALP is reported to be stable for 2-3 days refrigerated.
Interferences
- Lipemia/Turbidity, icterus: No significant inferences.
- Hemolysis: May increase ALP with severe hemolysis (>500 hemolysis index)
Test interpretation
Routine measurement of ALP gives total serum activity (all isoforms) without specificity as to source. In healthy animals, L-ALP is the predominant isoform in blood, followed by B-ALP. The proportion of B-ALP is higher in young animals (indicates osteoblastic activity with growth). The C-ALP isoform contributes only a small amount to total serum ALP activity. The proportion of C-ALP increases with age in dogs.
Isoform measurement is most commonly applied to canine samples to distinguish L-ALP and C-ALP isoforms in cases with increased total serum ALP activity of uncertain cause (to identify whether total ALP is increased due to liver disease or endogenous corticosteroids). However, this is not a very reliable test for this purpose, because any chronic disease (including that affecting the liver) can result in endogenous corticosteroid release (chronic stress), which would increase C-ALP.
We are most concerned about increases in ALP activity, however an unexpectedly low ALP activity (e.g. young growing animal) may be a marker of growth hormone deficiency (Bakhtiani and Burgener 2019), but is unlikely to be specific for this.
Increased ALP activity
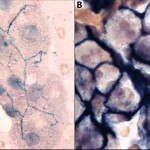
Increases in serum ALP activity are usually due to the liver, bone and corticosteroid-inducible isoforms (remember, dogs are the only species which have a steroid-inducible isoform). Therefore we attribute high ALP activity due to cholestasis, increased osteoblastic activity (not osteolysis) and steroids (dogs only). Increased ALP in serum or plasma due to increases in other isoforms are rare, likely because the enzyme gets released into the lumen of organs or has a very short half-life (see table above).
- Physiologic:
- Age: ALP activity in young, growing animals of all species may be 2-10 times higher than adults, due to the increased B-ALP isoform. Values decrease within 3 months of age and are within adult ranges by 15 months of age. Neonates also show increased ALP levels; this is believed to be either from high levels of ALP in colostrum or induced ALP production from actual colostrum ingestion.
- Breed of dog: Some Siberian Huskies have benign (transient) familial hyperphosphatasemia. This syndrome is characterized by high ALP activities (> 1100 U/L at 11 weeks of age and > 700 U/L at 16 weeks of age). It is not associated with any clinical effects and is due primarily to the bone isoform (Lawler et al 1996). High ALP activities have also been reported in Scottish Terriers, but this may be secondary to underlying subclinical adrenal dysfunction (the increase is due to C-ALP) (Zimmerman et al 2010, Cortright et al 2014; see more below), which may be a genetic-based defect in this breed.
- Endogeneous corticosteroid release (chronic stress): Initially, the ALP activity increase is due to the L-ALP isoform; the C-ALP isoform takes several days (up to 10) to be induced. This phenomenon only occurs in dogs.
- Drugs or hormones
- C-ALP (dogs only): This isoform is induced by exogenous corticosteroids (Solter et al 1994).
- L-ALP induction by drugs:
- Anticonvulsants (e.g. phenobarbital, primidone) have been reported to increase ALP activity, which was attributed to induction (stimulation of gene expression) (Müller et al 2000) but increases may be due to drug-induced hepatic injury instead.
- Corticosteroids will affect ALP activity to variable degrees in dogs, depending on the formulation or dose, with persistence of increases for up to 3 weeks after cessation of therapy with a single subcutaneous dose of 1.1 mg/kg methylprednisolone (Ginel et al 2002). In one study, treatment of dogs for 32 days with 4 mg/kg of prednisone initially increased L-ALP followed by C-ALP in 5-7 days (Sanecki et al 1987). Increases in ALP activity above reference intervals due to corticosteroid treatment have not been reported in other species. The increase in L-ALP after corticosteroids has been shown to be due to induction of synthesis (Wiedmeyer et al 2002).
- Pathophysiologic:
- Hepatobiliary disease: Increases in ALP (primarily the L-ALP isoform) is used as an indicator of cholestasis (intra- or extrahepatic, mostly structural) in animals. Bile acid accumulation within hepatocytes stimulates L-ALP production. In one study in 270 dogs with and without histologic evidence of liver disease, the highest ALP activities were seen in dogs with steroid hepatopathy, cholestasis (e.g bile duct obstruction), chronic hepatitis and hepatic necrosis (the latter could be due to concurrent localized structural or functional cholestasis) (Center et al 1992). In cats, ALP is considered a specific but insensitive marker of hepatobiliary disease, being less frequently increased versus ALT or AST in 54 cats with histologic evidence of liver disease (Center et al 1986). Increases in ALP activity do occur in hepatobiliary disease in cats, particularly hepatic lipidosis, cholangiohepatitis-cholangitis, extrahepatic bile duct obstruction, and liver tumors (e.g. lymphoma) but the increase is less reliable and of lower magnitude compared to analogous situations in dogs (Center et al 1986, Center et al 1992). The lower concentration of ALP in the cytoplasm of feline hepatocytes and the short half-life of this enzyme in the cat (6 hours) makes many clinicians feel small changes in ALP activity should not be ignored in cats (but do not always mean liver disease, since increased ALP activity can be seen with hyperthyoridism). The wide range of ALP activities and insensitivity of this test to cholestasis in large animals limits utility of ALP in these species. In horses, increases in total and direct bilirubin concentrations due to cholestasis may precede increases in ALP activity (personal observations). For this reason, ALP is no longer included on our large animal biochemical or liver panels.
- Structural cholestasis: This is due to a physical impediment to bile flow, which can be intrahepatic or extrahepatic (or a combination of both).
- Extrahepatic cholestasis (bile duct obstruction): This causes very dramatic increases in ALP. Increases in ALP may occur before development of icterus or increased total or direct bilirubin concentrations, especially in the dog or with localized cholestasis. Pancreatitis (acute or chronic) may result in increased ALP levels from swelling and/or fibrosis around the bile duct (especially in cats due to their common bile and pancreatic duct).
- Intrahepatic cholestasis: Localized or generalized cholestasis from hepatocyte swelling will induce ALP (we have seen cytologic evidence of c cholestasis, e.g. bile casts in liver aspirates, in dogs with increased ALP but not direct or total bilirubin concentrations). Lesions that are primarily centrilobular generally cause only mild increases in ALP while lesions affecting the periphery (periportal areas) of the lobule usually result in more dramatic elevations as a result of impaired bile flow. Causes of intrahepatic cholestasis include neoplasia (primary or metastatic), hepatic lipidosis (marked increases are possible with idiopathic hepatic lipidosis in cats – lipidosis is the cause of the most dramatic increases in ALP activity in this species, often without concurrent increases in GGT, which is a useful diagnostic feature), acute hepatocellular injury (intrahepatic cholestasis occurs due to hepatocellular swelling; increased ALT activities are expected concurrently in dogs and cats or SDH/GLDH in large animals), and periportal fibrosis and inflammation (marked increases are possible).
- Functional cholestasis: This is defined as decreased bile flow due to cytokine-mediated downregulation or inhibition of transporters responsible for excreting bile salts or conjugated bilirubin into bile. It occurs without any physical obstruction or impairment to bile flow. It has been reported in dogs with E. coli infections. It likely occurs in other species as well. Cytokine-mediated cholestasis is usually characterized by high total bilirubin (due to direct and indirect bilirubin) with mild increases in hepatocellular leakage enzymes (ALT, AST, SDH, GLDH). ALP activities may be normal or increased in this condition.
- Neoplasia: In primary liver cancer (hepatocellular/biliary), marked increases in ALP activity are possible (due to L-ALP or C-ALP in dogs). Metastatic neoplasia to the liver often increases ALP due to localized cholestasis. In many cases of hepatic neoplasia, ALP may be the only enzyme that is increased on a panel. In one study of 114 Scottish Terriers with adrenal dysfunction, vacuolar or copper-associated hepatopathy, ALP activity was not significantly different between those animals with and without hepatocellular carcinoma (Cortright et al 2014).
- Acute hepatocellular injury: As mentioned above, mild to moderate increases in ALP activity are attributed to intrahepatic cholestasis associated with hepatocellular swelling rather than hepatocellular injury per se. Concomitantly high values of ALT, SDH and GLDH would be expected.
- Structural cholestasis: This is due to a physical impediment to bile flow, which can be intrahepatic or extrahepatic (or a combination of both).
- Hyperadrenocorticism in dogs: Activites vary from moderate to marked (up to 100- fold) and are frequently due to induction of the C-ALP isoform (although L-ALP increases are also seen) in dogs. Up to 83-100% of dogs with Cushing’s disease have high C-ALP activity, but chronic endogenous stress (due to any underlying disease) may increase C-ALP and total serum ALP (up to 2-3 x normal). Therefore, C-ALP activity is a sensitive, but not specific, test for hyperadrenocorticism in dogs.
- Adrenal dysfunction in dogs: Adrenal disease, causing increases in sex hormones or cortisol secretion, is associated with increased ALP activity (as high as 22,000/uL) in Scottish Terriers (Cortright et al 2014).
- Non-hepatic neoplasia: Benign or malignant mammary tumors in dogs have been associated with increased ALP, irrespective of metastasis or the presence of osseous metaplasia. The increases in ALP activity are generally mild (median ALP activity of 170 U/L, ranging from around 50 to 480 U/L), with increases in bone, liver and corticosteroid isoforms (Karayannopoulou et al 2006). The ALP is possibly of myoepithelial cell origin. Dogs with osteosarcoma (tumor of osteoblasts) can have high ALP activity and it was a negative prognostic indicator, with an activity > 120 U/L being associated with a poor prognosis in some studies (Boerman et al 2012). The high ALP may be related to the proliferative nature of the neoplasm, however cell lines derived from patients with high or low serum activity showed no difference in proliferative rates in vitro (Holmes et al 2015).
- Increased osteoblastic activity (non-neoplastic): The osteoblastic activity in response to hormones (parathyroid hormone, thyroid hormone) may increase total serum ALP activity due to the B-ALP isoform. This can be seen in dogs with primary and secondary hyperparathyroidism (2-3x increase) and healing fractures and hyperthyroidism in cats. In dogs with healing long bone fractures, the mean ALP activity peaked around 360 U/L 10 days after surgery in dogs with radiographic evidence of good healing, whereas lower to no increases in ALP activity occurred in dogs with slower or non-healing fractures (mean around 260 U/L and 100 U/L activity, respectively), peak at 10 days (Komnenou et al 2005). Hyperthyroid cats may have mild increases in total serum ALP activity, which is mostly due to high B-ALP with a lesser increase in the L-ALP isoform (Archer and Taylor 1986). They may also have concurrent mild increases in ALT and AST, but not GGT, activities mimicking a pattern of enzyme changes seen with hepatic lipidosis. Successful treatment of hyperthyroidism results in significant decreases in ALP activity (Williams et al 2014).
- Hepatobiliary disease: Increases in ALP (primarily the L-ALP isoform) is used as an indicator of cholestasis (intra- or extrahepatic, mostly structural) in animals. Bile acid accumulation within hepatocytes stimulates L-ALP production. In one study in 270 dogs with and without histologic evidence of liver disease, the highest ALP activities were seen in dogs with steroid hepatopathy, cholestasis (e.g bile duct obstruction), chronic hepatitis and hepatic necrosis (the latter could be due to concurrent localized structural or functional cholestasis) (Center et al 1992). In cats, ALP is considered a specific but insensitive marker of hepatobiliary disease, being less frequently increased versus ALT or AST in 54 cats with histologic evidence of liver disease (Center et al 1986). Increases in ALP activity do occur in hepatobiliary disease in cats, particularly hepatic lipidosis, cholangiohepatitis-cholangitis, extrahepatic bile duct obstruction, and liver tumors (e.g. lymphoma) but the increase is less reliable and of lower magnitude compared to analogous situations in dogs (Center et al 1986, Center et al 1992). The lower concentration of ALP in the cytoplasm of feline hepatocytes and the short half-life of this enzyme in the cat (6 hours) makes many clinicians feel small changes in ALP activity should not be ignored in cats (but do not always mean liver disease, since increased ALP activity can be seen with hyperthyoridism). The wide range of ALP activities and insensitivity of this test to cholestasis in large animals limits utility of ALP in these species. In horses, increases in total and direct bilirubin concentrations due to cholestasis may precede increases in ALP activity (personal observations). For this reason, ALP is no longer included on our large animal biochemical or liver panels.
ALP/GGT patterns
Please refer to the GGT page for some tidbits on interpreting some changes in these two cholestatic enzymes.