Iron concentrations are measured as a way to assess iron stores in the body. However serum or plasma iron is not a good marker of body iron stores. This is because iron concentrations in blood change rapidly because iron is turned over constantly (frequently enters and exits cells) during normal metabolism and is strongly influenced by inflammatory cytokines and a protein called hepcidin. Rather, the best test (“gold standard”) for body iron stores is measurement of hepatic iron (which is rarely done in living animals as it is a quite invasive procedure to get a 1 g liver biopsy to measure hepatic iron). Subjective evaluation of bone marrow iron (with a Prussian blue stain, which detects hemosiderin within macrophages) can be used as a surrogate for liver iron, however this is also infrequently done for the diagnosis of iron deficiency anemia.
Measurement of serum iron concentrations is usually performed to help confirm a diagnosis of iron deficiency anemia. It is rarely used for diagnosis of iron excess (a very infrequent disorder in animals). At Cornell University, we include iron panels (iron, TIBC, % saturation) on our routine mammalian chemistry panels, because the results can provide useful adjunctive information in animals with or without anemia or iron deficiency. In other words, the results of an iron panel should never be interpreted alone, but always in the context of hemogram and other biochemical results and the patient’s clinical signs and known disease processes. For example, a low serum iron and % saturation in an animal with a microcytic hypochromic anemia supports an iron deficiency anemia, whereas a low iron and % saturation in an animal with a normocytic normochromic anemia (or without anemia) is likely due to causes other than iron deficiency, such as inflammation.
Note, that serum or plasma tests for iron are not measuring “free” iron (which is a strong free radical and would cause major tissue damage if truly “free”) or iron within hemoglobin in red blood cells. Rather it is measuring the iron that is bound to apotransferrin, the iron transport protein in blood. In fact, we measure serum or plasma iron by liberating it from transferrin, using low pH conditions (just as iron would be removed from transferrin within the phagolysosome of cells) (see below).
Physiology
Iron is taken up from dietary sources. If more iron is taken up then is lost from the intestine in normal daily use or used for hemoglobin synthesis or other cellular activities, the excess iron is stored within cells, specifically macrophages (most of their iron comes from red blood cell turnover) or hepatocytes. Iron within these cells can be released for use when needed, so these locations serve as body stores of iron. Iron stores do build up in our body and we need these stores to provide iron in states of nutritional iron deficiency or excess iron loss (usually from loss of blood, since RBC contain most of the iron within the body). Young animals have low stores of iron, because they need iron for growth and milk is low in iron, but iron stores build up quite quickly.
Once ingested from the intestine, iron is moved around the body bound to apotransferrin in plasma. Cells have receptors for transferrin and, when they need iron, transferrin receptors are unregulated on their surfaces and binds transferrin (apotransferrin + iron). The transferrin receptor-transferrin complex is internalized by clathrin-mediated endocytosis and, within the acidic pH of the phagolysosome, iron is liberated off transferrin. The transferrin receptor-apotransferrin is then recycled back to the surface; the apotransferrin is then freed and can go and pick up more iron from macrophages, hepatocytes or intestinal epithelial cells. Naturally, erythroid progenitors in the bone marrow have the most need for iron (for hemoglobin synthesis) and have the highest concentrations of transferrin receptor (this is lost as immature RBC lose protein synthetic capability as they mature). Iron is also recycled in the body. When RBC naturally age and reach the end of their normal lifespan, they are phagocytized and degraded by macrophages. The iron within their hemoglobin is released into the macrophage, which can store the iron (if iron is not needed), release the iron for use by erythroid progenitors (which cluster around macrophages in bone marrow, waiting for their iron) or release iron to transferrin for transport to other cells in the body. For a graphic illustration of this, please refer to the iron physiology page.
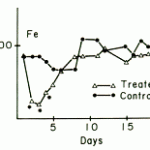
Thus, factors which affect iron concentrations are absorption, release and uptake by cells, red blood cell turnover, use (conversion to hemoglobin within erythroid progenitors) and loss. Iron stores are regulated principally by uptake from the intestine. There is no regulation of iron loss (sloughing, blood loss).
- Absorption: Dietary iron is normally absorbed in duodenum. Dietary iron is obtained either from inorganic sources (Fe3+) or animal sources (in heme from breakdown of hemoglobin or myoglobin). To be absorbed from the intestinal lumen, iron must be in the ferrous state (Fe2+). Inorganic sources of iron are converted to ferrous iron by an enzyme on the luminal (apical) surface of enterocytes. Iron then enters the cell through a transporter, called DMT-1 (divalent metal transporter-1). Heme within myoglobin or hemoglobin binds to an apical surface receptor called HCP-1 or heme carrier protein-1. The heme-HCP-1 complex is internalized by endocytosis and iron is released from the heme ring (iron + porphyrin) into the cell by an enzyme called heme oxygenase-1. Once within the cell, iron (regardless of dietary source) is stored as ferritin, used in enzymes as needed within the intestinal cell, or transferred to the basal membrane, where it exported into plasma via a transporter called ferroportin-1. Once in plasma, iron is taken up by apotransferrin, the iron transport protein. In order for apotransferrin to take up iron, it must be in the ferric state (Fe3+). However, within the intestinal cell, it is in the ferrous (Fe2+) state. A copper-dependent enzyme called hephaestin, which is located at the basolateral surface of intestinal cells, helps oxidize iron to the ferric state, allowing transfer from the intestinal cell into plasma. From there the iron bound to transferrin can be taken up by cells bearing transferrin receptors and used for various cellular processes, such as division. Absorption is influenced by the following:
- Dietary content: Low iron content will lead to low iron stores, e.g. milk is low in iron
- pH: Acid pH in the intestinal lumen decreases.
- Intestinal disease: May decrease absorption.
- Hepcidin: Decreases absorption by inhibiting uptake from the intestine. Hepcidin causes internalization and degradation of ferroportin-1, thus decreasing release from enterocytes.
- Vitamin C: Increases aborption by keeping iron in the ferrous state which helps iron to be taken up by DMT-1.
- Copper: Increases by promoting release from enterocytes (it is an essential cofactor for haephestin, which converts iron to the ferric state, allowing uptake of intestinal source iron by transferrin).
- Release and uptake from cells: This is influenced by various factors and results in rapid transient variations of iron concentrations, even in a normal subjects. There is normally a high iron turnover with only a tiny amount of this being reflected in blood levels, hence it is not surprising that iron values can vary tremendously within and between days in a single individual.
- Within day variation in iron: This has been identified in dogs and in one study of 9 research dogs (Miniature Schnauzers and Beagles housed in controlled lighting conditions), iron concentrations were associated with cortisone concentrations, with the mean iron and cortisol concentrations being lowest in the morning (7 am versus 3 pm and 11 pm, Harvey et al 1987). In another study, iron concentrations in 6 research Beagle dogs in controlled lighting were similar at 8 am and 12 am then lower at 4 and 8 pm. One dog in the latter study had a serum iron concentration near 100 ug/dL (close to the lower limit of the reference interval). Cortisone concentrations were not measured and information about stimuli that could promote cortisone release before the 8 am timepoint were not provided (Chikazawa et al 2013). TIBC concentrations in both studies remained relatively constant.
- Hepcidin: This peptide, produced in the liver, decreases iron rapidly by preventing release from macrophages, enterocytes and liver cells (this is called sequestration). This is because hepcidin causes internalization and degradation of ferroportin, the transporter that moves iron from within cells to transferrin, the transport protein in plasma. Decreased ferroportin will result in iron accumulation within cells and decreased serum iron concentrations, with the decrease in concentrations occurring quite rapidly (within several hours). Hepcidin values increase in response to increased iron stores and inflammatory cytokines.
- Inflammatory cytokines: Interleukins (particularly IL-6) and TNFα stimulate hepcidin synthesis, decreasing iron uptake from the intestinal tract and release from body stores in macrophages and hepatocytes. Cytokines also induce apoferritin synthesis resulting in sequestration of iron within macrophages (as ferritin). This decreases iron concentrations in plasma and increases iron stores. Increases in α1-antitrypsin (an acute phase reactant protein) will decrease binding of transferrin to transferrin receptors and decrease internalization of the transferrin receptor-transferin complex. This decreases iron availability to the red blood cells and iron release from macrophages. Intravenous infusion of purified lipopolysaccharide to horses (500 ng/BW dose) causes a rapid drop in serum iron concentrations (within 6 hours and stays low for 48 hours), likely due to inflammatory cytokine release. The drop in iron concentration preceded increases in serum amyloid A concentrations (Lillehöök et al 2020).
- Infectious agents (pathogen-associated molecular patterns) and toll-like receptor (TLR) signaling: Engagement of TLR2, 4, 6, 7/8, but not TLR 3, 5 and 9, causes intracellular iron sequestration in human THP-1 monocytic cells, via different mechanisms. TLR2 activation, such as by specific peptides or Listeria monocytogenes, causes downregulation of ferroportin transcription, whereas TLR4 ligands, like lipopolysaccharide, induce hepcidin transcription and translation. Attenuated Mycobacterium bovis, a TLR2 and 4 agonist, causes intracellular iron retention via downregulation of ferroportin and upregulation of hepcidin (Abreu et al 2018).
- RBC turnover: Increased RBC turnover, e.g. hemolytic anemia, ineffective erythropoiesis, may increase iron concentrations in plasma (this will be a transient effect).
- Decreased use (incorporation into hemoglobin): Inhibition of hemoglobin production, decreased erythropoiesis particularly due to pure red cell aplasia may result in decreased iron concentrations in plasma.
- Loss: Loss of iron from the body will deplete iron stores and result in iron deficiency anemia (eventually). Since RBC are the biggest repositories of iron, loss of iron is usually due to chronic external blood loss (e.g. from the gastrointestinal tract). Testing for fecal occult blood is a method to evaluate for such chronic blood loss. Note, that small amounts of bleeding or acute hemorrhage will not result in an iron deficiency anemia because iron can be released from stores. It is only when stores are depleted (with chronic blood loss) that iron deficiency results. Low iron concentrations in an acutely bleeding animal is more likely due to inflammatory cytokines or transient variation than it is due to iron deficiency from blood loss. Internal blood loss (e.g. hemoperitoneum) will not result in an iron deficiency because the iron is not “lost” – it is recycled within the body and reused.
Methodology
Reaction type
End point, blanked (an initial optical density measurement is taken before addition of reagent. This decreases the effect of interferences such as lipemia, hemolysis and icterus, which may falsely increase results without a sample blank)
Procedure
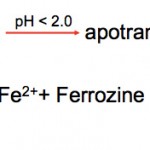
Serum or heparinized plasma iron represents the iron bound to apotransferrin. Binding is tight at physiological pH so the assay takes advantage of acidification (which normally liberates iron from transferrin inside cells within the acidic phagolysosome of macrophages) by citric acid and thiorurea to release the ferric iron from transferrin. Once released, the ferric iron is converted to ferrous iron by ascorbic acid (included in the reaction) and a color change is produced from the ferrous iron reacting with a specific substrate, with concentrations being expressed in µg/dL.
Units of measurement
Iron is measured in μg/dL (conventional units) or μmol/L (SI units). The conversion formula is shown below:
μg/dL x 0.179 = μmol/L
Sample considerations
Sample type
Serum, plasma, urine
Anticoagulant
Heparin is acceptable. Anticoagulants with chelating agents such as EDTA, oxalate, and fluoride should not be used (will result in falsely low concentrations).
Stability
Stable if separated from cells.
Interferences
- Lipemia: No known effect
- Hemolysis: May increase concentrations (due to liberation of iron from hemoglobin with a hemolysis index > 200 units (per our manufacturer’s application sheet). In our experience, there is no direct correlation between iron and the degree of hemolysis (hemolysis units), suggesting that this is not a major artifact. However, prolonged storage with hemolysis may increase iron concentrations.
- Icterus: No known effect
- Drugs: Corticosteroids will increase iron in dogs and horses. Dexamethasone decreases iron in cattle.
Test interpretation
Decreased concentrations (hypoferremia)
The most common causes of low iron are transient variation and sequestration secondary to inflammatory cytokines (which inhibit iron absorption and prevent iron release from macrophages through upregulating hepcidin). Compared to these two causes, true iron deficiency (from decreased intake/absorption or external blood loss) is a less common cause of low serum or plasma iron concentrations.
- Artifact: Contamination of sample with EDTA or other chelating anticoagulant.
- Physiologic: Random transient variations can occur in a healthy subject. Studies in humans have shown that iron concentrations can change rapidly in normal subjects with no change in total body iron. When iron is measured on consecutive days in individual patients, concentrations can vary from above to within to below the reference interval (in a random fashion) on the different days. Iron concentrations also fluctuate in dogs. In one study where iron and cortisol concentrations were measured at 3 time points during the day (7 am, 3 pm, 11 pm) for 3 days in dogs housed in controlled lighting conditions, iron and cortisol concentrations were lower in the morning. However values stayed within reference intervals (Harvey et al., 1987). In another study, the reverse was seen in that iron concentrations were higher in the morning than later in the day or evening (Chikazawa et al 2013; see above). Differences between these two studies could be related to housing conditions in relation to sampling. Some young animals (e.g. kittens between 2-4 weeks of age) can have low iron.
- Iatrogenic: Dexamethasone injections have been shown to decrease iron in cattle (as much as half) – the mechanism for this decrease is unknown.
- Pathophysiologic
- Decreased absorption or intake: Absorption, which occurs in the duodenum, is decreased by acidosis, hepcidin, copper deficiency (an essential cofactor of hephaestin, which permits release of iron from intestinal cells), zinc excess (inhibits copper uptake), inadequate dietary content (e.g. milk is low in iron) and intestinal disease. Copper deficiency or zinc excess can thus result in an iron deficiency anemia from decreased iron absorption as well as inadequate release from stores (since copper is also an essential cofactor for ceruloplasmin, which releases iron from macrophages) – this particularly occurs in herbivores. Malnutrition has been associated with low iron in cattle (mechanism is uncertain).
- Sequestration: Iron is sequestered within cells (typically iron storage cells such as macrophages or hepatocytes) in inflammatory or chronic disease states or (sometimes) portosystemic shunts. Inflammatory cytokine-mediated sequestration is the most common cause of low iron concentrations in both anemic and non-anemic animals but does not result in microcytic hypochromic red blood cells (like true iron deficiency).
- Mild trivial injury/trauma: Iron can decrease quickly even in relatively mild or acute injuries or trivial infections. This is likely inflammatory cytokine-mediated. The decrease in iron is often transient. Siderophores and lactoferrin will bind iron locally in areas of inflammation but will not result in low serum iron levels.
- Inflammation: Low iron is due to inflammatory cytokine-induced synthesis of ferritin and hepcidin. Inflammatory cytokines (particularly IL-6 and TNFα) stimulate apoferritin synthesis resulting in sequestration of iron within macrophages. This decreases iron concentrations in plasma and increases iron stores in cells. Low iron in serum or plasma is a sensitive marker of acute inflammation in horses (Borges et al., 2007). Similar findings have been observed in dogs (Torrente et al 2015), cattle (Baydar and Dabak 2014) and alpacas (Passler et al 2013). In one prospective study of 116 dogs admitted as emergencies, plasma iron concentrations were lowest in dogs with systemic inflammatory response syndrome or sepsis (64 ± 42 μg/dL) than dogs with localized inflammation (89 ± 40 μg/dL) or healthy blood donor controls (139 ± 40 μg/dL). The same study showed that measurement of plasma iron had good discriminatory ability for localized or systemic inflammation (versus blood donor controls; area under the curve ranging from 0.836 to 0.888 in receiver operating characteristic curves), which was similar to the performance of C-reactive protein (which is considered a highly sensitive marker of acute inflammation) and fibrinogen (Torrente et al 2015). In camelids, significantly decreased iron concentrations occurred between 12 and 36 hours after treatment with low-dose endotoxin (via an osmotic pump placed subcutaneously). Decreased concentrations of iron preceded increases in other acute phase proteins, including haptoglobin and serum amyloid A (which was not detected with the assays used in the study) (Passler et al 2013).
- Portosystemic shunts: Iron appears to be sequestered in hepatocytes in some animals, resulting in low iron stores, iron concentrations in serum or plasma and even red blood cell microcytosis (shunts infrequently results in anemia and hypochromic red blood cells). The mechanism is unknown.
- Cancer: Low iron concentrations are seen in some dogs with cancer. Loss of p53 (a tumor suppressor gene) has been associated with increased hepcidin concentrations, indicating that low iron in animals with hepcidin may be due to tumor-induced, and not cytokine-induced, secretion of hepcidin.
- Loss: Since much of the body’s iron stores are found in red blood cells (hemoglobin), chronic persistent external hemorrhage will result in iron deficiency, once stores within macrophages are depleted. Sources of loss include gastrointestinal, urinary, reproductive and respiratory tracts (e.g. epistaxis). Because iron is required for hemoglobin production, smaller red blood cells containing less hemoglobin are produced in states of iron deficiency – thus an iron deficiency anemia is recognized clinically as a microcytic hypochromic anemia. This may or may not be regenerative. The most common cause of iron deficiency anemia in adult animals is bleeding into the gastrointestinal tract. In older small animals, gastrointestinal neoplasia, intestinal parasites (e.g. hookworms, whipworms) and vascular ectasia or angiodysplasia (a condition associated with dilatation of large intestinal mucosal blood vessels, which causes chronic intestinal or colonic hemorrhage in dogs) are common causes of iron deficiency anemia, whereas Haemonchus contortus infestation of the C3 gastric compartment is a common cause of iron deficiency in camelids. Iron deficiency in horses and ruminants is unusual, although we have observed copper deficiency in ruminants (resulting in a secondary iron deficiency and microcytic anemia). Young animals, particularly those that are suckling, are predisposed to more rapid development of iron deficiency than adults, should they have an iron deficient diet or a source of blood loss (e.g. flea infestation), because milk is low in iron and they have low body iron stores.
Increased concentrations (hyperferremia)
The most common causes of increased iron in serum or plasma are transient or random variation, acute hepatocellular necrosis, corticosteroid therapy (dogs, horses) and increased iron turnover. Iron excess (overload) syndromes are rare in animals. There is some confusion with the terms related to syndromes of iron overload. Hemochromatosis is a term that should be restricted to inherited or primary causes of iron overload, e.g. defects in transporters of iron in the intestine resulting in increased iron uptake. The excess iron is found within macrophages and cells. The iron accumulation is pathologic and frequently results in hepatic cirrhosis or liver failure. Hemosiderosis, in contrast, is a secondary condition due to iron overload from parenteral administration of excess iron, blood transfusions etc. With this condition, iron accumulates in macrophages, not tissue cells. This is reversible and usually not pathologic.
- Artifact: Potentially hemolysis (see above)
- Physiologic: Random transient variation in normal subjects (see above). This is transient.
- Iatrogenic: Corticosteroid administration may increase iron in dogs and horses (up to 2x). In one study in dogs, serum iron values were increased from days 1 through 3 after administration of oral prednisolone to healthy beagle dogs at a dose of 2 mg/kg BID for 3 days (Harvey et al., 1987). Consistent changes in TIBC were not observed in the latter study. Even anti-inflammatory doses of corticosteroids (0.5 mg/kg BID) can increase iron concentrations, with values increasing within 2 days of initiation of treatment and staying increased for 2 days after cessation of treatment (Adamama-Moraitou et al., 2005). Recent iron administration (particularly injectable forms of iron) will also rapidly normalize serum or plasma concentrations and can transiently increase concentrations. This may be the reason why some animals with decreased erythropoiesis have high iron and % saturation. Note that repeated blood transfusions can result in iron overload (hemosiderosis).
- Pathophysiologic:
- Release from intracellular stores: The liver is one of the major storage sites of iron, where iron (in ferritin) is seen in Kupffer cells and in hepatocytes. Release of high concentrations of ferritin (from necrotizing acute liver injury) with measurement of ferritin-containing iron (Tietz et al 1996) can result in high serum iron concentrations (and 100% percent saturation). From data through our laboratory, we have found that high serum iron (and % saturation) can be a marker of hepatic necrosis in dogs and horses.
- Increased red blood cell turnover: Iron is turned over rapidly each day, as part of normal red blood cell turnover (destruction of effete red blood cells). If there is excessive red blood cell turnover in the peripheral blood or in the bone marrow, high iron levels (with high % transferrin saturation) may be seen. This can occur in hemolytic anemias or disordered/abnormal erythropoiesis, such as primary myelodysplasia and ineffective erythropoiesis of any cause (e.g. precursor-directed immune-mediated anemia or non-regenerative immune-mediated anemia).
- Decreased erythropoiesis: Since red blood cells are the main utilizers of iron (to make hemoglobin), decreased or absent erythroid progenitors in marrow may result in increased iron, e.g. pure red cell aplasia. However, the high iron and % saturation seen in some animals with these conditions may be transfusion-associated (see above under iatrogenic).
- Iron overload: Syndromes of iron overload are rare but have been reported in Mynah birds (Mete et al., 2005), lemurs (Glenn et al., 2006), and Salers or Saler-cross cattle (O’Toole et al., 2001). The defect in Mynah birds is thought to be secondary to increased uptake from the intestinal tract; the birds express high levels of the iron transporters, the divalent metal transporter (DMT1) and ferroportin-1 (Ireg1) in their intestine.