To detect an acid-base disturbance, both a blood gas analysis and chemistry panel should be performed. This combines traditional blood gas analysis (Henderson-Hasselback equation) and the strong ion approach (by evaluating the contributions of electrolytes, in particular). The blood gas analysis and chemistry panel provide information on most of the variables responsible for acid-base disturbances and the results should be used together for interpretation. Note also that other information should be used to determine the cause for acid-base disturbances, including clinical signs and examination findings (e.g. diarrhea may cause a metabolic acidosis), the hemogram (e.g. hypoxia from an anemia may cause a respiratory alkalosis), and diagnostic imaging (pulmonary disease may cause respiratory acid-base abnormalities). This section also provides more detailed information on individual test results, specifically anion gap and bicarbonate. Information on sodium and chloride (strong ions) are given under the electrolyte section of the site.
General rules
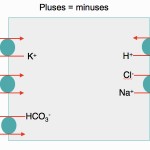
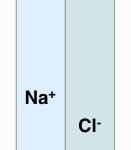
The body has protective mechanisms against acid-base disturbances and will attempt to compensate.
- Compensation opposes the primary disorder, i.e. compensation for a metabolic acidosis is a respiratory alkalosis.
- Compensation takes time
- Respiratory compensation for a metabolic disorder occurs within minutes.
- Metabolic compensation (usually via altering renal excretion) takes several days.
- Compensation does not usually return the blood pH to normal.
- An exception is chronic respiratory alkalosis and potentially chronic respiratory acidosis in dogs given sufficient time (weeks).
- Over-compensation does not occur.
- Sufficient time must be provided for compensation to occur.
- Electroneutrality rules: The body attempts to maintain electroneutrality at all times!
- This occurs in plasma, where the sum of positive charges = sum of negative charges (see gamblegram above).
- This occurs in cells, when they transport anions/cations across their membranes (see image above).
- Positive charges that dominate in blood: Sodium (Na+) > potassium (K+) > unmeasured cations (UC+), e.g. ionized magnesium, ionized calcium, γ-globulins.
- Negative charges that dominate in blood: Chloride (Cl–) > bicarbonate (HCO3–) > unmeasured anions (UA–). Note that unmeasured anions include negatively charged noncarbonic acids (e.g. phosphates, urates, hippurates, citrate), which dominate, and proteins, particularly albumin (this contributes less than acids to unmeasured anions).
- Do not over-interpret mild changes. No analyzer or test is 100% accurate!
Tests for acid-base disturbances
Identification of acid-base disturbances requires blood gas analysis and measurement of a chemistry panel, which includes the traditional assessment of blood gas as well as strong ions (electrolytes, in particular). All tests must be interpreted in the context of the patient. Examination of the patient should prompt consideration of underlying acid-base disturbances, e.g. an animal that is dyspneic could very well have a respiratory acid-base abnormality. The kidney plays an essential role in responding to and also generating acid-base disturbances and evaluation of renal function is imperative in most patients with acid-base abnormalities. These tests provide the following information:
- Blood gas analysis: pH (acidemia, alkalemia), respiratory component, metabolic component. Blood gas analysis may miss mixed acid-base disturbances and will not determine the nature of a metabolic acidosis (i.e. one due to consumption of bicarbonate or one due to loss of bicarbonate – a chemistry panel and assessment of the patient is required for the latter).
- Chemistry panel: This provides only the metabolic component and strong ion status (acid-base is influenced by changes in strong ions, particularly sodium and chloride). A chemistry panel can also identify the nature of a metabolic acidosis, because of the provision of an anion gap and electrolyte concentrations (sodium and chloride). A high anion gap metabolic acidosis indicates a titration acidosis or consumption of bicarbonate by accumulation of an acid or unmeasured anion. A normal anion gap hyperchloremic acidosis indicates a bicarbonate loss acidosis. These types of metabolic acidosis are seen in very different disorders in animals, so distinction between them is important. The chemistry panel gives no indication of pH or respiratory status.
Blood gas analysis
This can be performed on venous or arterial blood using a blood gas analyzer. Blood gas analysis is the only way we can determine the respiratory component of acid-base status and identify primary or secondary respiratory disturbances. The analysis provides the following information:
- pH: Directly measured.
- Respiratory component: partial pressure of carbon dioxide (pCO2), partial pressure of oxygen (pO2). These are directly measured.
- Metabolic component: Bicarbonate (HCO3–), total carbon dioxide (TCO2) and base excess (BE). These are calculated.
Interpretation of changes in each of these results is given below.
Some of the newer analyzers can also yield results for ionized calcium, acids (lactate) and oximetry parameters, e.g. saturation of oxygen.
Sample collection and handling
Blood should be collected from a peripheral artery or vein into syringes in which sodium (or lithium) heparin has been used to coat the syringe and needle (evacuated syringe) or specialized heparin syringes, which are electrolyte-balanced and have the correct amount of heparin and ideal for ionized calcium measurement. The latter syringes are preferred, but they are expensive, hence most people use the evacuation method. This is usually fine, however you should ensure that there is <5% heparin in relation to the volume of blood collected in the syringe. This is because excess heparin can influence blood gas values. The sample should be kept anaerobic at all times and analyzed promptly after collection to minimize false changes that occur with storage. Thus, blood gas analysis is a point of care test and a sample should not be sent by mail to a diagnostic laboratory for testing.
pH: Overall effect of disturbances
This is best evaluated in arterial blood gas samples, but can be done on venous blood gas samples (a lower pH would be expected in a venous blood gas due to acids, particularly lactate, generated by normal or abnormal tissue metabolism). This is directly measured by a pH electrode.
- Low pH = acidemia
- High pH = alkalemia
Note that the pH can be normal (within reference intervals) and there can still be acid-base disturbances (a primary disturbance which has not shifted the pH outside reference intervals or a mixed disturbance). Variables affecting pH results are:
- Falsely decreased: Excess heparin, EDTA, oxalate or citrate anticoagulants (only heparin should be used), sample storage (anaerobic metabolism by cells in the tube generate lactate)
- Falsely increased: Exposure to air (loss of carbon dioxide will increase pH).
Partial pressure of carbon dioxide: Respiratory component
This is the only laboratory indication of the respiratory component of acid-base status. Therefore, blood gas analysis is essential to evaluate the respiratory contribution to an acid-base disturbance. This is slightly higher in venous than arterial samples. This is directly measured using a carbon dioxide electrode.
- Low pCO2 (hypocapnea) = respiratory alkalosis
- High pCO2 (hypercapnea) = respiratory acidosis
The following variables can falsely decrease pCO2: Excess heparin, exposure to or mixing with air (10% air bubbles will affect results), aged samples.
Partial pressure of oxygen: Oxygen status
This test result is only reliable in arterial blood samples because tissue consumption of oxygen will automatically decrease the pO2. It indicates the amount of dissolved oxygen in the blood sample. This is directly measured using an oxygen electrode.
- Low pO2 = hypoxemia
- High pO2 = hyperoxemia. Hyperoxemia does not usually occur naturally but can be seen in animals given supplemental oxygen (usually during anesthesia).
Bicarbonate: Metabolic component
The bicarbonate, with the total carbon dioxide and base excess, is an indicator of the metabolic component of acid-base status. It is a calculated value and reported as the standard bicarbonate, which is the concentration of bicarbonate in the plasma after equilibration with carbon dioxide at a partial pressure of 40 mm Hg. It is calculated from the pH and pCO2, based on the Henderson-Hasselbach equation (where pH = 6.1 = log10(bicarbonate ÷ [0.03 x pCO2] ).
- Low HCO3– = metabolic acidosis
- High HCO3– = metabolic alkalosis
Variables that falsely decrease bicarbonate are: Prolonged venous stasis (prompts anaerobic metabolism), excess heparin (>10% of collected blood volume), sample storage (lactate consumes bicarbonate). For more on interpretation of bicarbonate, see the bicarbonate page (which pertains to bicarbonate measurement on the chemistry panel).
Total carbon dioxide: Metabolic component
The total carbon dioxide (TCO2) is equivalent to HCO3– + dissolved CO2 (dissolved CO2 = pCO2 x 0.03, which is the solubility coefficient of carbon dioxide). Much of the dissolved CO2 is lost to the atmosphere after collection so the TCO2 is usually equivalent to or 1-2 mEq/L higher than the HCO3–. This is a calculated value (as shown above).
- Low TCO2 = metabolic acidosis
- High TCO2 = metabolic alkalosis
This can be falsely decreased by storage (lactate consumes bicarbonate and dissolved carbon dioxide is lost to air). Collection into EDTA can falsely increase values. Bicarbonate is a dependent variable and will be directly affected by changes in pCO2 (when dissolved in blood or plasma water) based on the following formula:
CO2 + H20 ↔ H2C03 ↔ H+ + HCo3–
Base excess (BE): Metabolic component
The base excess is the amount of strong acid or base required to titrate 1 liter of blood to pH 7.4 whilst pCO2 is kept constant at 40 mmHg. It is calculated from the hematocrit, pH and pCO2. Base excess is affected by changes in non-volatile (or noncarbonic) acids and is thus an indication of the metabolic component of acid-base status. This is considered to be the best indicator of the metabolic component of acid-base, since unlike bicarbonate, it is not influenced directly by changes in carbon dioxide (see above). However, in reality, the bicarbonate, total carbon dioxide and base excess change in parallel and can be interpreted similarly.
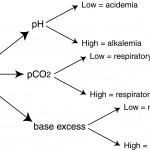
- Low BE = insufficient base or metabolic acidosis
- High BE = excess of base or metabolic alkalosis
A buffer is a molecule which can accept a positively charged hydrogen ion (H+). The whole blood buffer base is the sum of all buffers in the body. These buffers are found in the vascular compartment (bicarbonate, proteins with negative charges, phosphates) and within cells (phosphates and proteins, particularly hemoglobin in red blood cells). The most important buffer for acids generated in the body (metabolic acidosis) is bicarbonate, whereas intracellular buffers are important for buffering carbon dioxide (respiratory acidosis). This is because carbon dioxide generates bicarbonate and hydrogen when it dissolves in plasma water (see above equation). Thus, bicarbonate cannot titrate a respiratory acidosis or primary increase in carbon dioxide.
The above are the typical results obtained from a blood gas analyzer. However, what this analysis does not provide is information on the type of metabolic acidosis (consumption of bicarbonate or bicarbonate loss) and strong ion levels (sodium, chloride). Without measurement of strong ions and calculation of the anion gap (AG), the type of acidosis cannot be determined and a mixed disturbance may be missed.
Chemistry panel
This can be performed on serum or heparinized plasma and results are optimal if the sample is analyzed fresh (to prevent lactate accumulation from anaerobic glycolysis of cells in vitro) with minimal exposure to air. The chemistry panel provides information on metabolic disturbances only; blood gas analysis is essential for identifying respiratory disturbances and determining if the patient is acidemic or alkalemic.
The important aspects of the chemistry panel for assessment of acid-base status are:
- Electrolytes: Na+, K+, Cl–. Sodium and chloride are strong ions, which affects acid-base balance independently. Potassium impacts the ability of the kidney to respond to a acid-base abnormalities. The strong ion approach to acid-base relies heavily on interpretation of electrolytes and how they influence acid-base status. This is good because changes in these electrolytes dictate treatment of an acid-base disturbance due to strong ion changes.
- Bicarbonate: Bicarbonate is measured differently than the blood gas analyzer, but is interpreted similarly.
- Anion gap (AG): The anion gap is the sum of unmeasured anions minus the sum of unmeasured cations and is essentially equivalent to unmeasured anions (which outnumber unmeasured cations in health and disease).
- Minerals: Ionized calcium, ionized magnesium, inorganic phosphate. These behave as unmeasured anions (phosphate, ionized calcium) and unmeasured cations (ionized magnesium). Phosphate has the largest effect on acid-base status.
- Proteins: Albumin and globulins. At physiological pH, these behave as unmeasured anions (albumin) and cations (immunoglobulins).
- Renal function: Urea nitrogen, creatinine.
How these tests are used to help identify acid-base disturbances are given below.
Electrolytes
- Sodium: Na+ is the most abundant cation in the body and is also a strong ion. It may be helpful to think of sodium as a base.
- High Na+: An increase in sodium is compatible with an alkalosis (strong ion approach)
- Low Na+: A decrease indicates an acidosis (strong ion approach).
- Chloride: Chloride is the most abundant anion in the body and is a strong ion. Its concentration should always be assessed in relation to changes in sodium (since they circulate together), otherwise an acid-base disorder will be missed. It would be helpful to think of chloride as an acid.
- Proportionate changes in chloride (with respect to sodium): Na+ and Cl– will change proportionally when there are disturbances (loss or gain) of free water or concomitant losses of both electrolytes.
- Disproportionate changes in chloride (with respect to sodium): A change in chloride that is disproportionate to the change in sodium is not due to changes in free water and indicates an acid-base disturbance. Therefore, Cl– values should always be interpreted with Na+. Indeed, Cl– can be “corrected” for changes in Na+ to identify a disproportionate gain or loss of Cl– and, thus, an underlying acid-base disorder. This can be done in two ways:
-
- Eyeballing the data (assessing the Na+ and Cl– results to determine if the changes are in the same direction and roughly similar in relation to the upper or lower limits of the reference intervals;
- Calculating a corrected chloride, using the following formula:
Corrected chloride (Cl–corr) = (Na+(average)/ Na+(measured)) x Cl–(measured)
where, average = midpoint of reference interval; measured = actual value obtained
Since chloride is an acid, disproportionate changes in chloride are interpreted as follows:
Low Cl–corr = Metabolic alkalosis (loss of chloride which is a strong anion/acid; this is usually accompanied by high HCO3–).
High Cl–corr = Metabolic acidosis (gain of chloride which is a strong anion/acid; this is usually accompanied by low HCO3–).
- Potassium: This is a cation and may affect acid-base status indirectly, by affecting the ability of the kidney to excrete or retain acids. For example, a low K+ potentiates a metabolic alkalosis by promoting renal acid excretion in the kidneys. In rare cases, a severe hypokalemia can actually be the primary cause of a metabolic alkalosis, e.g. primary hyperaldosteronism (this is because K+ moves out of cells in exchange for H+, to maintain electroneutrality. The movement of H+ intracellularly decreases H+ in blood, resulting in a metabolic alkalosis). Potassium is also used (by some) in the anion gap calculation.
Bicarbonate
The HCO3– is measured directly with the chemistry analyzer and results should be similar to that obtained from a blood gas analysis (if the sample is handled correctly). Note that a normal HCO3– does not rule out an acid-base disturbance. In mixed disturbances, there can be opposing effects on the HCO3– (metabolic alkalosis will increase, whereas metabolic acidosis will decrease, HCO3–), so the HCO3– may be normal. In this instance, it is essential to evaluate Cl–corr and AG results, otherwise a mixed disturbance will be missed (see more information on mixed acid-base disturbances below). Changes in bicarbonate are interpreted as follows (assuming correct sample handling):
- Low HCO3– = Metabolic acidosis
- High HCO3– = Metabolic alkalosis
Anion gap (AG)
The anion gap is an important result that helps distinguish the type of metabolic acidosis. The anion gap is a calculated test using the following formula:
AG = Na+ + K+ + UC = Cl– + HCO3– + UA,
where UC = unmeasured cations and UA = unmeasured anions.
Rearranging the formula, you get:
AG = (Na+ + K+) – (Cl– + HCO3–) = UA – UC.
So, in reality, the anion gap should be thought of as the difference between unmeasured anions (which outnumber cations) and unmeasured cations and not as a calculation of sodium, potassium, chloride and bicarbonate. Note that some people do exclude K+ from the calculation, but this is not recommended, particular in cattle where potassium concentrations can be quite low (Constable 2014). Also, the measured and not the corrected chloride is used in this calculation.
- Unmeasured anions: Endogenous acids (lactate, ketones, phosphate, sulfates, urate, hippurate, citrate), metabolites of toxic or drug metabolites (ethylene glycol, methanol, salicylic acid) or proteins (primarily albumin). As can be seen, “unmeasured” is somewhat of a misnomer, because phosphate is measured on a panel, but it is not included in the AG calculation (therefore, it is “unmeasured”).
- Unmeasured cations: Minerals (ionized versions; Ca2+, Mg2+, Zn2+, Mn2+) and proteins (γ-globulins). See comment above for “unmeasured”.
In essence, the AG is equivalent to the difference between unmeasured anions and cations. Since unmeasured anions are more abundant than cations, the AG is usually positive and can be assumed to be an indicator of unmeasured anions. Note, that since albumin and globulins (particularly IgG) are unmeasured anions and cations at physiologic pH, changes in these proteins will influence the anion gap. For example, dehydration masking a hypoalbuminemia may actually falsely increase the anion gap. Changes in the anion gap indicate the following:
- Low AG = Decreased unmeasured anions or increased unmeasured cations (rare). Since protein (particularly albumin) are unmeasured anions, a decrease in anion gap can be seen with hypoalbuminemia. A decrease in AG does not provide much additional information, by itself, on acid-base status. In fact, a low AG can indicate an error in measurement of the AG components, e.g. bromide will falsely increase Cl– without altering Na+, thus decreasing AG.
- High AG + low HCO3– = Increase in unmeasured anions. This usually indicates a primary metabolic acidosis, specifically a titration metabolic acidoss (HCO3– is titrating or being consumed by an unmeasured anion, i.e it is acting as a buffer). Acids only accumulate in the body in pathologic states, e.g. renal failure, lactic acidosis.
- Normal anion gap + low HCO3– = No change in unmeasured anions or cations. This usually indicates a bicarbonate loss metabolic acidosis (this can be primary or secondary to a primary respiratory alkalosis), which is accompanied by a high corrected chloride (loss of HCO3– is accompanied by a gain in Cl–; remember electroneutrality rules!).
Proteins
Since albumin is an unmeasured anion and immunoglobulins are more cationic than anionic at physiological pH (7.4), these will affect the AG and changes in these proteins should be considered when interpreting the AG. Changes in protein values have less of an impact on the AG compared to the strong ions. Protein results are used more in the strong ion approach to acid-base status. For more information on these protein measurements, see related links below.
Minerals
Since inorganic phosphate is an unmeasured anion and ionized Ca and Mg are unmeasured cations, these will affect the AG and changes in these results should be considered when interpreting the AG. Phosphate is a strong ion and is used more in the strong ion approach to acid-base status. Since ionized calcium and magnesium are found in relatively low concentrations in health or disease, they contribute only a little to changes in the anion gap and are not considered strong cations. For more information on these minerals, see related links below.
Renal tests
The kidney not only has a central role in responding to acid-base disturbances, but can also be responsible for a primary disturbance (or lack of compensation or attempted correction) if kidney function is abnormal. For example, renal azotemia (and sometimes post-renal azotemia) may cause a titration (high anion gap) acidosis, due to decreased renal excretion of acids normally filtered through the kidneys, e.g. phosphates, sulfates and citrates (remember, high phosphate is a feature of decreased glomerular filtration rate). Therefore, results of renal tests should be assessed to determine the potential cause of a metabolic acid-base disturbance. Furthermore, a normally functioning kidney is required for the body to compensate for a primary respiratory disturbance or to help correct a primary metabolic disturbance. Note, that not all renal disease will result in acid-base abnormalities. An animal can have renal disease, but still be able to compensate for a respiratory acid-base disturbance or may not have a primary metabolic disturbance due to the renal disease. For more information on renal function, see related links below.
Guidelines for interpretation
As indicated above, at Cornell University, we combine traditional blood gas interpretation (looking at pH, bicarbonate or base excess and pCO2), with strong ions (electrolytes and the anion gap, in particular). This will help identify the majority of disturbances that occur in animals versus looking at blood gas alone. This approach does include, to a degree, the contribution of protein and other minerals (phosphate) on blood gas analysis, but these have less of an effect on acid-base balance than strong ions. The blood gas analysis provides pH, bicarbonate or base excess (metabolic component) and pCO2 (respiratory component) but not the contribution of strong ions (sodium and chloride in particular), protein (albumin, especially) or minerals (phosphate). The latter is obtained from the chemistry panel. Here we give one approach to interpreting blood gas and chemistry results that are related to acid-base balance.
Blood gas analysis
- Look at the pH first.
- If abnormal, there is an acid-base disturbance (as long as the sample was collected and stored properly). For example, an animal has a low pH, a high pCO2 and high bicarbonate or base excess. The low pH indicates an acidemia, which must be explained (primary respiratory or primary metabolic – so you know how to treat the patient).
- However, if the pH is not abnormal, do not stop there – still look at the rest of the results, as indicated below, otherwise you will miss a mixed disturbance (in which the pH is frequently normal, but individual test results are not).
- Find an explanation for the pH changes: What is the primary acid-base disturbance that is explaining the change in pH?
- Look at the respiratory (pCO2) component: Is this abnormal and does the abnormality explain the change in pH? In the above example with a low pH and high pCO2, the high pCO2 indicates a respiratory acidosis (and is going in the right direction) hence the acidemia is likely due to animal to a primary respiratory acidosis (primary because it is explaining the change in pH).
- Look at the patient to explain the disturbance (does this make sense?). In the above example, does the animal have tracheal collapse? In the case above, the animal did indeed have tracheal collapse, which would more than adequately explain a respiratory acidosis.
- Look at the metabolic (bicarbonate, base excess) component: Is this abnormal and does the abnormality explain the change in pH? In the above example where the pH is low and pCO2 is high, the bicarbonate or base excess is high. This does not explain the low pH since the bicarbonate is going in the wrong direction (up = metabolic alkalosis versus down = metabolic acidosis; the latter would explain a low pH but the former would not). Thus, we cannot attribute the low pH to a metabolic acidosis.
- Look at the patient to explain the disturbance (does this make sense?).
- If the pH is normal and there are still changes in and bicarbonate, determining which is the primary disturbance (there is likely more than one) is difficult. This requires looking at the patient to see what disorders would explain the changes in blood gas results and evaluating the degree of change, e.g. if the pH is on the lower end of the reference interval, acidosis is likely dominating (but the animal is not acidemic because the pH is not low).
- Is there a compensatory response? Once you have decided which is the primary disturbance (the result that is changed in the correct direction, considering the changes in pH), assess if there is a compensatory response. In the example above, we have a primary respiratory acidosis in an animal with tracheal collapse. The expected compensatory response is a metabolic alkalosis and the bicarbonate and base excess are high, supporting a compensatory response. We do not expect compensation to normalize the pH so this makes sense. But, we could possibly have a concurrent primary metabolic alkalosis. How do we make sure the bicarbonate and base excess mean a compensatory response (with excretion of chloride, an acid, by the kidney to compensate for the decrease in blood pH) or a concurrent primary metabolic alkalosis that is not as severe as the primary respiratory acidosis?
- Look at the patient! The dog with tracheal collapse has no evidence of a primary disease abnormality or process resulting in loss of an acid or gain of bicarbonate, e.g. vomiting gastric contents (rich in hydrochloric acid or loss of an acid).
- Look at the chemistry results: Kidney function was normal (the kidney is essential for responding to or compensating for a primary respiratory acid-base disturbance) and there was a high bicarbonate with a low corrected chloride (supporting loss of chloride through the kidneys). Thus, the high bicarbonate and base excess indicate that the kidney was appropriately compensating for the high pCO2 (respiratory acidosis) by excreting chloride (as ammonium chloride or NH4Cl).
- Lastly, look at the patient to see that your interpretations are valid.
Chemistry panel results
- Look at the bicarbonate first.
- If abnormal, there is likely an acid-base disturbance (as long as the sample was collected and stored properly).
- However, if the bicarbonate is not abnormal, do not stop there – still look at the rest of the results, as indicated below, otherwise you will miss a mixed disturbance.
- Look at the anion gap (AG): Is this high? If the anion gap is high, there is an increase in unmeasured anions and bicarbonate is being consumed (or is titrating or being consumed by an accumulated acid). This indicates a titration acidosis due to accumulation of a noncarbonic acid, e.g. lactate.
- Look at the patient to determine what acid is accumulating, e.g. is this a diabetic cat with accumulating ketoacids?
- Look at protein values because these are unmeasured anions (albumin) and cations (globulins, particularly γ-globulins)
- Look at renal results, because kidney function affects acid-base. Indeed, a renal azotemia can lead to a high anion gap acidosis due to retention of acids by the failing kidneys (phosphates, hippurates, citrates).
- Look at and correct the chloride for changes in sodium: Is the corrected chloride abnormal and what abnormality is present? (remember, do not overinterpret mild changes.) In the example above of a diabetic cat accumulating ketoacids, we would expect the corrected chloride to be abnormal. However, the cat may be concurrently losing HCl (an acid) through vomiting gastric contents (this can occur in diabetes mellitus). This would create a concurrent metabolic alkalosis, which may affect the bicarbonate results (and even normalize them, hence a normal bicarbonate on a chemistry panel does not rule out an acid-base disturbance. If there are changes in corrected chloride and anion gap, there is likely a mixed metabolic disturbance present).
- Look at the patient to explain the disturbance (does this make sense?).
- Assess the contribution of protein (albumin especially) and phosphate.
- Take a blood gas to assess for pH changes and primary and compensatory respiratory disturbances.
- Lastly, look at the patient to make sure your interpretations are valid.
Related links
- Electrolytes: Measurement and interpretation of test results for sodium, potassium, chloride.
- Minerals: Measurement and interpretation of test results for calcium, magnesium, phosphate.
- Protein: Measurement and interpretation of test results for total protein, albumin and globulins.
- Renal function: Assessment of renal function, including physiology and measurement and interpretation of test results.
- Clinical Pathology Laboratory in the Animal Health Diagnostic Center at Cornell University: Information on blood-gas analysis that can be performed.