Chemical constituents that are a part of a urinalysis and included on a dipstick measurement: pH, protein, glucose, ketones, bilirubin and heme. We do not generally use the leukocyte and urobilinogen pads on the dipstick as they are considered inaccurate (dogs and cats at any rate for the leukocyte pad) or not diagnostically useful (urobilinogen). The stick can either be dipped into the urine (dip method) or the urine can be placed on each pad of the dipstick with a pipette (drip method) (for more on this, see the overview page).
In the case of urine protein, we can assess it in ways other than the dipstick. This includes using a sulfosalicylic acid precipitation test (not used much anymore), heat precipitation for Bence-Jones proteins or free immunoglobulin light chain (not used much anymore) and urine electrophoresis, which is used to detect light chains of immunoglobulins primarily, but also give us a “feel” for what proteins are in the urine (this is more accurately done with polyacrylamide gel electrophoresis than standard serum protein gel or capillary zone electrophoresis).
A more accurate measurement of protein in the urine is the protein to creatinine ratio. This measures the amount of protein in a spot sample of urine that is standardized to the creatinine concentration. Creatinine is a good marker for this purpose, because it is a small molecule that is freely filtered by the glomerulus and is typically not added to the urine or removed from the urine by the tubules, i.e. no resorption or secretion. This means, once filtered, its concentration remains fairly static in the urine, which makes it a good standardization marker for protein content. We perform this quantification or use this ratio when we are concerned about proteinuria that is excess for the urine specific gravity and want to determine if the excess proteinuria is due to tubular or glomerular dysfunction (see more below under protein to creatinine ratio).
Dipstick use
How do we use the dipstick analysis?
- Protein and glucose give us information on tubular function, specifically the ability of proximal tubules to resorb normally filtered low molecular weight substances such as smaller proteins and glucose. Proteinuria that is excessive for the USG and glucosuria without hyperglycemia can reflect proximal tubular disease (yes, other factors affect these as well as you will see below). In contrast, glomerular function is usually assessed by measurement of urea nitrogen and creatinine in serum or plasma. With a dipstick (or urinalysis in general), the only marker of glomerular function can be proteinuria that is excessive for the urine specific gravity. The proteinuria from glomerular disease is mostly albumin (around 68 kd) with increasing leakage of higher molecular weight proteins as barrier permeability is altered more and more with progressive disease, whereas the proteinuria with tubular disease is due to lack of resorption of normally filtered low molecular weight proteins (<68 kd). Naturally, we cannot distinguish between tubular and glomerular proteinuria on the dipstick protein result alone, but the urine protein to creatinine ratio (a more quantitative measurement of protein in urine) can be helpful (see more below).
- The other constituents on the dipstick do not usually tell us renal function per se but rather give us information on other pathologic processes occurring in the animal.
- pH: Although not very accurate, this tells us how the kidney is responding to acid-base disturbances or dealing with the normal acid load produced from amino acid metabolism. In some animals, pH can also tell us about renal tubular disease (e.g. in distal renal tubular acidosis, the pH of the urine is alkaline as the distal tubules can no longer acidify the urine or excrete acid, resulting in a systemic metabolic acidosis).
- Bilirubin (confirmed by an icto-test): Mostly gives information on the liver, particularly cholestasis, when present in the urine of any species (or excessive for the urine specific gravity in a dog, since a small amount of bilirubin may be seen in the urine of dogs).
- Ketones (when not a false positive): Indicates production of excess ketones as a result of negative energy balance.
- Glucose: Usually indicates overload (stress hyperglycemia, diabetes mellitus) but can indicate tubular dysfunction as indicated above.
- Heme; also called blood on the strip: Reacts with a heme group (porphyrin ring plus iron). Tells us there are red blood cells (hemoglobin from the red blood cells react) or free hemoglobin or myoglobin in the urine. The most common cause of a positive heme reaction is red blood cells in the urine (from collection or pathology).
pH
Knowledge of the urine pH is important in interpreting urine sediment findings. Erythrocytes, leukocytes, and casts tend to disintegrate in alkaline urine (pH > 8.0). In addition, precipitation of urine crystals in supersaturated urine is highly dependent on urine pH (e.g. struvite will precipitate in alkaline not acidic urine). The reportable range of pH by the CLINITEK Advantus Urine Chemistry Analyzer used at Cornell University is from 5.0 to >9.0, in 0.5 unit increments.
The table below illustrates the reportable pH results from the CLINITEK Advantus Urine Chemistry Analyzer used at Cornell University.
pH | |
5.0
|
7.5
|
5.5 | 8.0 |
6.0 | 8.5 |
6.5 | ≥ 9.0 |
7.0 |
Factors affecting the pH of urine
- Inherent variability of the method: Studies have shown that the dipstick measurement of urine pH can be quite inaccurate (e.g. a pH value of 7.5 can be anywhere from 7 to 8) (Johnson et al 2007). The pH showed the lowest level of agreement (>0.5 unit change) of all dipstick chemical constituents between observers (Boag et al 2019).
- Diet: Diet has a marked effect on urine pH. Grazing animals (herbivores) generally have alkaline urine except for young animals on a milk diet, where urine pH is more likely to be acidic. In contrast, carnivorous animals (dogs and cats) tend to have more acidic urine than adult herbivores, except straight after eating (called the post-prandial alkaline tide, due to increased secretion of HCl into the stomach). In one study, the pH of urine from dairy and beef cows ranged from 7.0-8.7 using graded strips for analysis (Herman et al 2018).
- Renal hydrogen (H+) excretion and bicarbonate (HCO3–) resorption
- Pathologic abnormalities of systemic acid/base balance.
- Pathologic abnormalities of tubular function: Failure to excrete an acid load (e.g. H+ excretion in distal tubules) or failure to absorb bicarbonate in the proximal tubules.
- Age of urine specimen: Over time, loss of CO2 to the air occurs, raising the urine pH.
- Presence of contaminant or pathogenic bacteria: Some bacteria can alter the pH of urine. Urease-positive bacteria such as Streptococcus, Ureaplasma or Proteus spp. convert urea to ammonia, which will increase pH. A persistently alkaline urine in a dog or cat should prompt testing for bacterial infection, which can precipitate stone formation due to alterations in pH (ammonium magnesium phosphate crystals are more likely to precipitate at alkaline than acidic pH, hence the use of acidifying diets to treat struvite urolithiasis).
Although the kidneys play a central role in the control of acid/base metabolism, the pH of a random urine sample is not a reliable indicator of total body acid/base status. In some conditions, impaired renal tubular function causes or perpetuates the underlying acid/base derangement. Meaningful evaluation of acid-base status generally requires blood gas analysis and consideration of clinical signs.
Protein: Dipstick
The protein pad on the multireagent dipstick is based on the “protein error of pH indicator dyes”. Basically, the test is dependent on the ability of amino groups in proteins to bind to and alter the color of acid-base indicators, even though the pH is unchanged. The reaction is more sensitive to albumin (as it contains the most amino groups) than globulins. It is insensitive to Bence-Jones proteins or free light chains (monoclonal or polyclonal) of immunoglobulins. Generally this differential sensitivity is not a substantial problem (most cases of significant proteinuria involve albuminuria).
The table below illustrates the reportable protein results from the CLINITEK Advantus Urine Chemistry Analyzer used at Cornell University.
Protein |
Neg
|
Trace |
1+ (30 mg/dL) |
2+ (100 mg/dL) |
3+ (>300 mg/dL) |
Interpretation
The presence of protein in the urine can yield clues as to the presence of glomerular barrier dysfunction (altered permeability) and proximal tubular disease. In fact, proteinuria can be an early marker of renal disease, even before the development of defects in urine concentrating ability or azotemia. However, this is disease dependent as some renal diseases, such as acute kidney injury, may not cause proteinuria at all.
The glomerular fluid is a filtrate of blood, with the glomerulus being permeable to proteins, based on molecular weight, size, shape and electrical charge of the protein molecules. It is also influenced by renal hemodynamics. The glomerular barrier is negatively charged so proteins that are more cationic (positively charged) at physiologic pH (such as albumin) are more likely to be able to enter urine or are more freely filtered than more negatively charged proteins. A major influence of whether proteins will be normally filtered by the glomerulus is the molecular weight of the protein. The renal threshold is around 68,000 daltons, therefore the glomerular filtrate contains electrolytes (in a similar concentration to plasma) and low molecular weight proteins (<68 kd), such as β2 microglobulin. With a normal functioning kidney and tubules, these normally filtered small molecular weight proteins are taken up and catabolized by the proximal renal tubular epithelial cells resulting in little to no protein in the urine. Albumin has a molecular weight close to 68 kD and is more positively than negatively charged at physiologic pH. Therefore, only very small amounts of albumin can be seen in urine from healthy animals without renal disease. When we do see proteinuria, we want to think about where it is coming from and, with respect to renal disease or function, we think of two main conditions:
- Glomerular barrier alterations: Alterations in the glomerular barrier from changes in renal blood flow (e.g. passive venous congestion in congestive heart failure, exercise) may result in mild proteinuria, which is often termed as a “functional” proteinuria, because it is not thought to be clinically relevant and can be transient. In contrast, glomerular disease will result in more substantial proteinuria or a pathologic glomerular proteinuria. The proteinuria initially consists mostly of albumin and is called selective because only selective proteins of lower molecular weight (around 68 kD or slightly higher, i.e. around the same size as albumin) are allowed through the leaky glomerular barrier. As glomerular injury progressive, more and more protein comes through including higher molecular weight proteins and the proteinuria becomes non-selective and quite severe. Thus, severe proteinuria is typically due to glomerular disease.
- Tubular disease: Because the tubules resorb normally filtered proteins of low molecular weight (< 68 kd), tubular disease can result in a proteinuria if the tubules are not resorbing the freely filtered protein properly. Protein is normally resorbed in the proximal tubules so a tubular proteinuria (i.e. that due to tubular disease) indicates proximal tubule dysfunction. Because we do not filter a lot of low molecular weight proteins normally, the proteinuria from tubular disease is milder than that due to glomerular disease. If a patient with renal disease has glomerular disease that allows more protein through from the beginning, tubular disease will worsen the proteinuria. In addition, albumin in the filtered urine fluid injures the renal tubules. When albumin is filtered in excess due to glomerular disease, the resorbed albumin causes an interstitial tubulonephritis and secondary tubular disease. Thus animals with glomerular disease can have concurrent tubular disease and one goal of treating animals with glomerular proteinuria, e.g. nephrotic syndrome (which is due to glomerular disease) is to try and reduce the albuminuria to help protect the tubules and kidney from more injury.
Thus, we use protein in the urine as a biomarker or indicator of renal disease, either affecting the glomerulus or tubules or both. Importantly, when we interpret protein in urine, we must take into account how concentrated the urine is, by concurrently assessing the urine specific gravity. Normal urine contains little protein; negative to trace reactions are usual in concentrated urine (urine specific gravity > 1.025), although rare stronger reactions for protein (up to 2+) can be seen in some animals, particularly dogs, with higher urine specific gravities (>1.030). Dilute urine (USG < 1.015) should have no protein in the urine, so even a trace to 1+ reaction in a dilute urine indicates excessive protein in the urine and we should look for a cause. A dipstick reaction > 2+ for protein in concentrated or dilute urine indicates significant proteinuria. Note that the dipstick protein only provides a subjective measurement of the degree of proteinuria. A more accurate assessment of proteinuria is obtained with measurement of the urine protein to creatinine ratio (UP:UC, see below), which standardizes the protein in the urine to creatinine, which remains constant in the tubules once filtered (not resorbed by the tubules or secreted by the tubules in most species). The dipstick measurement is also altered by the pH and you may see a false increase in protein in an alkaline urine (pH>8), but this is uncommon in our experience.
Although we use proteinuria as a biomarker of glomerular or tubular disease, there can be other reasons for excessive protein in the urine (i.e. protein excessive for the degree of concentration of the urine). These reasons include:
- The presence of small molecular weight proteins that are in excess in plasma that are then automatically filtered into the urine and exceed the ability of the renal tubules to absorb them. This is called a preglomerular, prerenal or overload proteinuria because the kidney is being overloaded by these proteins. An example is high concentration of free hemoglobin in plasma from a hemolytic anemia with an intravascular hemolytic component that then is filtered into the urine. For more, see below.
- Urinary or reproductive tract inflammation and hemorrhage: These conditions may also result in proteinuria because of the of the plasma proteins (not cells) that is coming along with the red blood cells (with hemorrhage) or due to protein exudation (with inflammation or bacterial infection) . Note that reproductive tract inflammation or hemorrhage is more likely to influence protein values in a free catch urine or urine obtained by catheterization versus that obtained by cystocentesis.
Thus, in animals with these conditions (overload, genitourinary inflammation or hemorrhage), it is very difficult to know if there is concurrent glomerular or tubular injury, which is what we are looking for when using a dipstick to measure protein in urine (they are confounding variables).
More information about these different categories or types of proteinuria is given below.
When we see proteinuria it is good to think about all the sources for protein in the urine and we do this by separating proteinuria into categories (Lees et al 2005):
- False proteinuria: Alkaline urine (pH of 8 or greater) can sometimes result in a false positive result for protein in the urine with the dipstick. This is uncommon in our experience, but always possible.
- Pre-renal proteinuria: Pre-renal proteinuria (also called overload or pre-glomerular proteinuria) occurs when there are large concentrations of small proteins which have molecular weights (MW) below the normal glomerular threshold, e.g. colostral proteins, Bence-Jones proteins (free light chain of immunoglobulins), hemoglobin monomers or dimers and myoglobin. These proteins are all normally <68 kD (around 16-22 kD) so will be freely filtered by the glomerulus. Thus, when in excess in plasma, they will be present in excess in the glomerular filtrate and are not able to be completely resorbed by the renal tubules, resulting in proteinuria (they exceed the absorptive capacity or overload the tubules). A colostral proteinuria occurs in neonatal animals less than 40 hours old. Excess free light chains occur in animals with plasma cell neoplasia, including extramedullary plasmacytoma and multiple myeloma. Hemoglobinuria will occur in states of intravascular hemolysis. There should be concurrent hemoglobinemia and red urine supernatant (if there is large amounts of hemoglobin) with an anemia if there is a proteinuria due to hemoglobinuria. Myoglobin will leak into the urine in conditions of severe muscle injury (rhabdomyolysis). Increased activities of muscle enzymes (CK, AST) will be seen in chemistry panels from affected animals, although the urine may not be discolored unless the myoglobinuria is severe. Note that both hemoglobin and myoglobin will cause a positive result for heme (“blood”) on the dipstick, but the protein reaction on the dipstick is less sensitive to hemoglobin and myoglobin than it is to albumin.
- Renal proteinuria: Renal proteinuria occurs when there are alterations in glomerular permeability (glomerular proteinuria) or inability of the tubules to resorb small molecular weight (MW) proteins which are normally filtered into the tubules through the glomerulus. A form of renal proteinuria also occurs when disease processes within the kidney cause leakage of serum protein into the urine, e.g. renal hemorrhage or inflammation (this used to be called post-renal proteinuria and is now called interstitial proteinuria when it is occurring within the kidney versus lower down the urinary tract).
- Glomerular proteinuria: Glomerular proteinuria can be functional or pathological.
- Functional proteinuria: results in a mild proteinuria and is due to increased hydrostatic pressure or an altered glomerular filtration coefficient, e.g. stress, exercise, fever, excitement, congestive heart failure.
- Pathological glomerular proteinuria: This is due to renal disease (e.g. renal amyloidosis, glomerulonephritis) and can be selective (resulting in loss of small MW proteins such as albumin) or non-selective (with loss of larger MW proteins, such as globulins) through the glomerulus in excess of the tubules ability to resorb them (in this case, there are no tubular abnormalities, at least initially). With glomerular disease, the proteinuria usually starts off as selective, with only albumin being lost (due to its small size). As the disease worsens, the glomerular filter barrier is further disrupted and higher MW proteins leak into the urine, resulting in a non-selective proteinuria. The dipstick result is usually 2+ or greater and excessive for the USG in pathologic glomerular proteinuria. The urine protein:creatinine ratio (UP:UC) is usually > 2 and often > 5 in dogs. This can result in hypoalbuminemia if urinary albumin loss is severe or prolonged. A good example of a disease causing glomerular proteinuria is renal amyloidosis where amyloid deposits in the glomeruli causing a leaky or dysfunctional barrier. Animals can develop nephrotic syndrome (proteinuria in excess for the urine specific gravity, hypoalbuminemia from urinary protein loss, and hypercholesterolemia from production of low density lipoproteins [mechanism unclear]. These animals can have concurrent edema but are not always azotemic.
- Tubular proteinuria: Tubular proteinuria is due to decreased proximal renal tubule function resulting in decreased absorption of filtered low MW proteins or increased excretion/leakage of proteins by damaged proximal tubules. This results in a mild to moderate proteinuria, i.e. dipstick readings of 2+ or less and UP:UC usually < 2 in dogs, and consists of low MW proteins with only small amounts of albumin. This does not result in hypoalbuminemia (you are not losing albumin excess). Causes of tubular malfunction are many and varied, e.g. Fanconi syndrome, renal ischemia, nephrotoxins (such as aminoglycosides). In cases of tubular proteinuria, there can be other evidence of tubular malfunction in the urine sediment, e.g. inappropriate USG, cylindriuria, glucosuria (without hyperglycemia).
- Interstitial proteinuria: Occurs from altered vascular permeability, hemorrhage or inflammation within the kidney.
- Glomerular proteinuria: Glomerular proteinuria can be functional or pathological.
- Hemorrhage/inflammation: As indicated above, interstitial proteinuria is the term used to describe hemorrhage or inflammation at the level of the kidney. Post-renal proteinuria is due to hemorrhage or inflammation in the urinary tract (ureter, bladder, urethra) or in the reproductive tract that causes protein (in the plasma that accompanies inflammation or hemorrhage, not within the cells themselves) to enter the urine once it has been formed and entered the renal pelvis.
- Inflammation: Inflammation and/or infection anywhere in the distal urinary or genital tracts, e.g. cystitis (including feline interstitial cystitis [Panboon et al 2017]), prostatitis, metritis, will cause proteinuria from leakage of serum protein along with leukocytes or due to increased vascular permeability. The UP:UC is an unreliable indicator of urinary protein loss in the presence of inflammation or infection (i.e. an active urine sediment).
- Hemorrhage: In severe hemorrhage, the dipstick pad will be unreadable due to the urine color. Hemorrhage frequently results in proteinuria when serum protein accompanies erythrocytes. Hemorrhage can occur anywhere within the urogenital tract (including the kidney itself, although proteinuria associated with renal hemorrhage would be called renal interstitial proteinuria) but more frequently reflects lower urinary tract (bladder disorders) or reproductive tract disease. Note that traumatic microscopic hematuria associated with cystocentesis does not usually result in proteinuria (personal observations, Vaden et al 2004) or a UP:UC >0.5 (based on spiking canine urine with peripheral blood [Vaden et al 2004, Jillings et al 2019]), although there are reports of UP:UC >0.5 in dogs and >0.4 in cats in dark yellow spiked urine (Vientós-Plotts et al 2017)
False positive results
- Alkaline urine: False positives occur rarely in highly buffered or alkaline urine samples as the citrate buffer is overcome, resulting in a pH shift. Titration of the sample to a more neutral pH and retesting could be done to overcome this problem, but is not routinely performed. The sulfosalicylic acid (SSA) precipitation test used to be performed as an alternative, however several studies have shown that the urine dipstick meaurement of protein is reasonably accurate in most urine, even those that are alkaline, and the SSA reaction is no longer a necessary part of routine urinalysis
- Contact time: Leaching of the citrate buffer occurs if the urine remains in contact with the pad for a long time.
- Detergents: Quaternary ammonium compounds and chlorhexidine can result in false positives.
False negative results
- Bence-Jones proteinuria: A positive SSA protein reaction, with a negative or weak dipstick protein reaction, in a dog or cat with a high index of suspicion for multiple myeloma, is suggestive of the presence of free light chains in the urine. This is because the dipstick is less sensitive to free light chains of immunoglobulins but the SSA will precipitate these proteins.
Protein: SSA
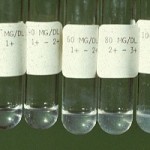
Protein in urine can also be estimated using sulfosalicylic acid (SSA) precipitation. The SSA reagent is added to a small volume of urine. Acidification causes precipitation of protein in the sample (seen as increasing turbidity), which is subjectively graded as trace, 1+, 2+, 3+ or 4+. Unlike the protein test on the dipstick, the SSA reaction will detect albumin and globulins (although it is more sensitive to albumin). In addition, the SSA detects Bence-Jones proteins (which are usually not picked up by the dipstick), although it often underestimates them.
In the past, the SSA reaction was used to confirm positive reactions for protein on the dipstick in alkaline urine. However, studies have shown that the SSA reaction (which is also a highly subjective assessment) is no more accurate (and in fact can be less accurate) than the dipstick for measurement of urine protein. For this reason, the SSA reaction is no longer routinely performed on urine samples at Cornell University. If there is concern that the urine protein on the dipstick is falsely increased in an alkaline urine sample, measurement of urine protein (or protein to urine creatinine ratio) on a chemistry analyzer is advised.
False positives
- Contrast media
- Antibiotics in high concentration, e.g. penicillin and cephalosporin derivatives
- Uncentrifuged turbid urines can look positive. Therefore, SSA should always be performed on urine supernatant.
False negatives
- Highly buffered alkaline urine. The urine may require acidification to a pH of 7.0 before performing the SSA test.
- Dilute urine
- Turbid urine – may mask a positive reaction.
Protein: Bence-Jones
Bence-Jones proteins are free light chains of immunoglobulins – in reality, they can be polyclonal or monoclonal, although the term usually is applied to monoclonal light chains associated with plasma cell dyscrasias (e.g. multiple myeloma). (Remember that immunoglobulins are composed of two different types of polypeptide chains, heavy and light. Heavy chains determine immunoglobulin class, whereas light chains are common amongst the classes and consist of lambda and kappa chains).
Free polyclonal light chains (i.e. consisting of both lambda and kappa chains) are produced by all plasma cells. A small amount of these polyclonal light chains may be observed in urine from healthy patients and are increased if there is tubular dysfunction (free light chains have molecular weights < 44,000 daltons and are filtered by the glomeruli and catabolized by proximal renal tubule cells). In plasma cell disorders, such as multiple myeloma, free light chains may be produced in excess and are monoclonal in nature (either kappa or lambda, not both). These free monoclonal light chains are generally referred to as Bence-Jones proteins (B-J) and appear in the urine readily, because they are so small. They can be detected in urine by heat precipitation or urine electrophoresis.
Heat precipitation
Bence-Jones proteins display unique solubility characteristics. As urine is heated, they precipitate at approximately 56°C. They dissolve at 100°C. When urine is cooled, they re-precipitate at 56°C. In theory, this should work quite well. However, in practice, many patients with myeloma have renal dysfunction (myeloma kidney) and have albuminuria. Albumin precipitates at 100°C and does not re-dissolve, complicating interpretation of the test. Furthermore, not all canine or feline patients have urinary B-J (approximately 10-40% of canine myeloma patients have urinary B-J).
Urine electrophoresis
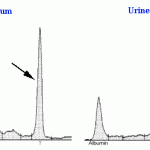
Bence-Jones proteins can sometimes be detected on urinary electrophoretograms, which should always be interpreted in conjunction with a serum electrophoretogram. Monoclonal B-J proteins are detected by the presence of a monoclonal peak in urine that mimics that in serum (see image below). Also, monoclonal B-J frequently migrate in the β-region of the electrophoretogram (whereas the paraprotein in serum may migrate in the β- or γ-regions). Once again, interpretation is confounded by albuminuria as a monoclonal peak in urine could represent heavy chain as well as light chain. If albuminuria is present but mild (<30 mg/dL), then the monoclonal peak in urine is likely free light chain (because the glomerular barrier is still selective in that albumin is mostly being retained in serum). In human patients, B-J proteinuria is confirmed by immunofixation using specific light chain antisera to confirm monoclonality. Unfortunately, suitable reagents are not available for veterinary patients to be able to perform this test. See total protein electrophoresis for more information.
Remember that urinary dipsticks do not detect B-J proteins very well, but sulfosalicylic acid will precipitate B-J as well as other urinary proteins.
Protein to creatinine ratio
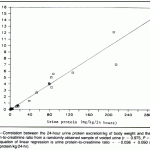
Urine protein to creatinine ratios are usually performed in animals in which there is excessive proteinuria for the urine specific gravity (see above), typically 2+ or more in a concentrated urine or 1+ or more in a less concentrated or more dilute urine. We perform this analysis to provide a more quantitative estimate of the amount of protein in the urine versus the semi-quantitative measure provided by a dipstick analysis. Essentially, what we are looking for this test is evidence for glomerular or tubular proteinuria or a combination of both, i.e. evidence of renal dysfunction in the glomerulus or the tubules that is resulting in proteinuria. We do not use this test if we are suspected a preglomerular or overload proteinuria or if there is interstitial proteinuria, e.g. spill over of light chain of immunoglobulins in an animal with multiple myeloma (overload) or post-renal proteinuria from hemorrhage or inflammation (interstitial proteinuria). This is because a more quantitative result for the amount of protein in the urine will not tell us about glomerular barrier or tubular function in patients with the latter conditions, because interpretation is confounded by the overload or protein accompanying hematuria or pyuria, respectively. Thus, this test should not be performed in animals with active urine sediments, i.e. those with pyuria, hematuria or bacteriuria. However, if you are concerned about glomerular barrier alterations or tubular dysfunction in a patient with hematuria or pyuria, you can retest the urine after the condition causing the hematuria or pyuria has been rectified. A persistent proteinuria that is excessive for the urine specific gravity in an animal without ongoing urinary inflammation or hemorrhage would definitely warrant a protein to urine creatine ratio measurement.
We measure protein in relation to creatinine concentration to account for the degree of concentration in the urine and standardize the protein concentration. Creatinine is a good standard in most settings because it is freely filtered by the glomerulus but is not resorbed or secreted by the tubules, i.e. once filtered by the glomerulus, it remains relatively constant (this is not true for goats, where the tubules can secrete creatinine into the urine). In reality, the most accurate method of urine protein quantification is to measure how much protein is excreted in the urine over 24 hours. But this is naturally not feasible in many patients and the “spot” test urine protein:creatinine ratio (UP:UC) on random mid-day urine samples correlates well to the 24-hour urine collection for quantitating urinary protein loss. It is unaffected by urine volume or concentration, i.e. takes into account how much water remaining within the tubules.
Unlike the dipstick, in which protein measurement is based on the change in color of a pH-sensitive dye (see above), for the urine protein to creatinine ratio (UP:UC), protein is quantified on a chemistry analyzer, which is more accurate than using the dipstick. There are several methods for measuring urine protein (and creatinine), including dye-binding (Coomassie brilliant B, pyrogallol red-molybdate) or turbidometric (e.g. benzethonium chloride, trichloroacetic acid, sulfosalicylic acid) methods. We use the latter method, which incubates the sample in EDTA at an alkaline pH (to denature protein and remove magnesium ion interference per product manufacturer sheet) before addition of the benzethonium chloride in a blanked end-point reaction.
Urine protein to creatinine ratios were stable for 4 hours at room temperature, 12 hours at 4°C and 72 hours frozen at -20°C in urine samples from 10 dogs with urine protein to creatinine ratios of 0.8-26.6 in glass or polymer-based containers (Moyle et al 2018).
Interpretation
Guidelines for dogs and cats are from ACVIM consensus statement (Lees et al., 2005):
- Dogs:
- Normal results: < 0.5 in healthy dogs without renal disease, non-azotemic dogs with renal disease or azotemic dogs, i.e. glomerular or tubular dysfunction resulting in excessive proteinuria is not evident (however, as indicated, animals can still have renal disease!).
- Equivocal results: 0.5-1.0 in non-azotemic dogs are considered equivocal. If concerned about renal causes of proteinuria (glomerular or tubular disease) or progressive non-azotemic renal disease, the consensus statement recommends continued monitoring for evidence of an increase in UP:UC.
- Abnormal: Values >1.0 in dogs without azotemia and values >0.4 in dogs with azotemia are abnormal and evidence for tubular or glomerular proteinuria and/or both. We use the degree of increase in the UP:UC as a guide to help distinguish between damage to the glomerular barrier causing proteinuria (glomerular proteinuria) or tubular injury resulting in proteinuria (tubular proteinuria). In general, tubular proteinuria is thought to result in milder proteinuria. Glomerular injury starts of with milder proteinuria (only smaller proteins like albumin getting through) but as it progresses, it becomes more severe. Thus, a severe proteinuria indicates glomerular injury. However, even in the latter cases tubular injury can be concurrent, but we need to look at other data to support this (e.g. granular, cellular or waxy casts; glucosuria without hyperglycemia).
- UP:UC > 1.0 and <2 in non-azotemic dogs: Proteinuria is thought to be mostly tubular with less of a glomerular component.
- UP:UC > 0.4 and <2 in azotemic dogs: Proteinuria is thought to be mostly tubular with less of a glomerular component.
- UP:UC ≥2 in non-azotemic or azotemic dogs: This is considered compatible with glomerular proteinuria, e.g. glomerulonephritis or amyloidosis. The severity of proteinuria does not distinguish between causes of glomerular disease, although dogs with renal amyloidosis frequently have the highest UP:UC (>10). Tubular proteinuria could also be present in animals with UP:UC >2.
- Note that these values are only valid for urine samples with inactive sediments.
- The consensus statement does recommend that azotemic dogs with UP:UC > 0.4 are treated for the renal disease causing the proteinuria, e.g. ACE inhibitors.
- Cats: The interpretation is the same as dogs. However, some healthy male cats without renal disease can have UP:UC results up to 0.6.
- Dairy/beef cattle: The UP:UC ranged from 0.05-0.25 in dairy cattle and from 0.04-0.14 in beef cattle in one study where the urine was catheterized (Herman et al 2018).
Factors affecting UP: UC independently of glomerular barrier leakage or tubular dysfunction
- Hemorrhage: The UP:UC will increase roughly proportionally to the degree of blood in the urine (due to the contribution of serum protein that comes along with the blood). Heavy blood contamination (>5%, i.e. red urine) with accompanying plasma protein may increase the UP:UC to >2.0 in individual dogs (Jillings et al 2019). Mild blood contamination from cystocentesis (i.e. 5-20 RBC/HPF or even > 100 RBC/HPF in a urine of normal color, i.e. microscopic hematuria) does not usually cause a proteinuria (on the dipstick or with urine protein to creatinine ratios) in our experience. Spiking canine urine with various amounts of peripheral blood (in dogs with serum protein <7.6 g/dL) to yield microscopic hematuria did not increase the UP:UC>0.5 in one study (Jillings et al 2019), whereas another study that spiked pooled urine samples from dogs and cats with various amounts of blood found a UP:UC >0.5 in dog urine and >0.4 in cat urine when the urine was dark yellow or light to dark yellow in a few pooled urine samples from these two species, respectively, whereas grossly visible hematuria (even when mild) did increase the UP:UC greater than these cut-offs in around 25-50% of the samples (Vientós-Plotts et al 2017).
- Infection: The UP:UC may not be accurate in the presence of a urinary tract infection. Ratios as high as 40 can be seen with E. coli infections in dogs. The ratio does not correlate to the number of red or white cells/HPF in these cases. Some cases of urinary tract infections can have normal protein to creatinine ratios (and no protein on the dipstick) so the degree of proteinuria in these cases cannot be predicted from the degree of cystitis. Proteinuria with infection is attributed to leakage of serum protein due to inflammatory-induced changes in vascular permeability which may depend on the severity and nature of the inflammation.
- Inflammation: Inflammation, without infection, may increase the UP:UC, but usually the ratio is < 2.0. In one study of 19 cats with idiopathic interstitial cystitis, the average UP:UC was 0.7 versus 0.1 in healthy cats and two cats with interstitial cystitis had UP:UC >2.0 (it is unclear how many of these cats had concurrent pyuria or hematuria, which was seen in these cats) (Panboon et al 2017).
- Therefore, urine protein-creatinine ratios should not be measured in animals with “active” urine sediments, as stated above.
- Drugs: Immunosuppressive doses of corticosteroids can increase the urine protein-creatinine ratio mildly in dogs, e.g. up to 1.3 with 2 mg/kg q 12 h for 6 weeks or by 20-34% at 2-4 mg/kg/d for 5 days, with higher % increases seen at 4 versus 2 mg/kg/d in dogs (Tinklenberg et al 2020). An increase in the urine protein-creatinine ratio was also seen in research beagles given 2 mg/kg prednisone for 28 days (increased from a median of 0.3 (range, 0.1-1.5) to 0.6 (range, 0.2-4.1) (Whittemore et al 2019). The reason for the increase is unclear, but it has been attributed to mesangial cell proliferation.
- Assay variability: Studies with dye-binding methods have shown variable coefficient of variation (CV, ranging from 6-8% for intra-assay to 10-11% for inter-assay) for urine protein measurement, with additional variability (albeit lower) for creatinine, resulting in an intra-assay CV ranging from 7-10.4% (higher for pyrogallol red-molybdate) and an inter-assay CV of 16-18% (higher for Coomassie Brilliant B) in cat urine. This variability resulted in a 10-45% altered classification rate based on International Renal Interest Society (IRIS) staging (Giraldi et al 2018). Changes were seen in urinary creatinine with frozen storage, although the authors stated that the UPC did not change significantly (data not shown for evaluation). Differences in the UPC were seen comparing the two dye-binding methods but one was done after frozen storage. This study indicates that not all UPCs are created equal and method of protein (and creatinine analysis) will influence the results. The bottom line is that for serial testing, the same laboratory (ideally with known method) should be used and switching between laboratories may not yield equivalent results.
Urine gel electrophoresis
Proteinuria due to tubular injury can be distinguished from proteinuria due to glomerular injury by performing gel electrophoresis on the urine. This is done using a sodium-dodecyl-sulfate-polyacrylamide gel electrophoresis (SDS-PAGE) or SDS-agarose gel electrophoresis. The SDS imparts a uniform charge to the proteins such they migrate different lengths in an electric current based on size and not charge (unlike standard serum or urine protein electrophoresis, which is done without SDS and using an agarose or cellulose acetate gel). Normal canine urine contains a faint albumin band at around 55 kD, a faint band at 100 kD corresponding to Tamm-Horsfall protein, and 3 bands below 54 kD in intact male dogs due to proteins associated with the prostate. Tubular proteinuria is characterized by the presence of increased amounts of low molecular weight proteins, below the glomerular molecular weight cut-off in the urine (<54 kD), such as retinol binding protein (21 kD). Glomerular proteinuria is associated with increased amounts of albumin and higher molecular weight proteins. The severity of the banding can be scored subjectively (Hokamp et al 2018). In one study of dogs with chronic kidney disease and proteinuria, a tubular but not a glomerular proteinuria pattern weakly correlated with tubular injury on light or electron microscopy, including tubulointerstitial fibrosis or chronic inflammation and tubular atrophy or degeneration. In contrast, glomerular and tubular proteinuria patterns were seen with light microscopic evidence of glomerular damage and the strongest correlation was seen with a glomerular pattern and electron microscopic evidence of glomerular injury. Tubular patterns in dogs with evidence of glomerular injury may be due to co-existence of tubular and glomerular injury (absorption of excess albumin can lead to chronic interstitial nephritis or tubular injury) or overload of tubular absorptive capacities with severe glomerular proteinuria (Hokamp et al 2018).
Glucose
Glucose is measured on the Multistix® by a glucose oxidase method. The reaction of glucose with glucose oxidase forms nascent oxygen (O), which converts potassium iodide in the dipstick pad to iodine, forming a brown color change. Normal urinary glucose is usually below the level of sensitivity of dipstick techniques, although a few animals may have trace positive reactions on the dipstick and small amounts of glucose in the urine when measured on a chemistry analyzer (Zeugswetter and Schewndenwein 2020). Therefore, glucose is usually an abnormal finding in urine (particularly when higher than trace). As always, a positive reaction for glucose on a dipstick should be interpreted in conjunction with the biochemical results (looking for hyperglycemia) and other evidence of tubular dysfunction (e.g. isosthenuric or inadequately concentrated urine in an azotemic animal, proteinuria excessive for the urine specific gravity, etc).
The table below illustrates the reportable glucose results from the CLINITEK Advantus Urine Chemistry Analyzer used at Cornell University.
Glucose (mg/dL) |
Negative |
100 (Trace) |
250 (Small) |
500 (Moderate) |
>1000 (Large) |
False positive reactions
- The presence of hydrogen peroxide, bacterial peroxidases (e.g. cystitis), hypochlorite and chlorine will produce false positive reactions.
- Formaldehyde
- Outdated reagents
False negative reactions
- High concentrations of ascorbic acid inhibit the reaction
- Drugs: salicylates, tetracyclines
Pathologic glucosuria
- Hyperglycemia (overload hyperglycemia): In many cases, glucosuria is a result of prior and often continuing hyperglycemia, to a level in excess of the renal threshold for reabsorption (transporters for glucose are found in the proximal tubules and are sodium-dependent – see glucose handling under renal physiology). The threshold in camelids is not known but is likely similar to the ruminant and horse.
Species | Threshold |
Canine | 180-200 mg/dL1 |
Feline | 2702-290 mg/dL* |
Bovine | 100-140 mg/dL |
Equine | 160-180 mg/dL |
* Diabetic cats can have lower thresholds (200 mg/dL). |
-
- Persistent hyperglycemia: Glucosuria is seen in diabetes mellitus, hyperadrenocorticism, acromegaly, and phaechromocytoma. Remember that glucosuria may mildly falsely increase the USG, because glucose contributes to the refractive index.
- Transient hyperglycemia: Stress-related hyperglycemia above the renal threshold has the potential to result in glucosuria. This is especially true in cats, camelid and cattle, which develop marked stress-related hyperglycemias that are over the rrenal threshold. Thus, the finding of glucosuria is not necessarily diagnostic for diabetes mellitus (in fact diabetes mellitus is very rare in cattle and camelids). Other conditions which produce transient hyperglycemia, e.g. pancreatitis, can potentially induce a mild, transient glucosuria, if blood glucose concentrations exceed the renal threshold. Studies have also shown a postprandial hyperglycemia and glucosuria in veal calves (Vicari et al 2008). A severe glucosuria (>1000 mg/dL), however, is suspicious for underlying diabetes mellitus.
- Abnormal proximal renal tubule function: Glucose is absorbed by a carrier-mediated process in the proximal renal tubules. Abnormal tubular function can result in glucosuria without hyperglycemia, but this is less common than overload, particularly with the aforementioned species.
- Physiologic: Young puppies (< 8 weeks old) can have mild glucosuria due to tubule immaturity.
- Renal tubule damage: This could be due to drugs (aminoglycosides), hypoxia, infections (Clostridium perfringens type D in sheep “pulpy kidney”) or other causes (e.g. proximal renal tubular acidosis which can be secondary to copper toxicity or idiopathic Fanconi syndrome [Cesbron et al 2017]).
- Inherited renal diseases: Primary renal glucosuria, Fanconi syndrome, etc.
Ketones
The ketone pad on the multi-reagent dip stick detects mainly acetoacetic acid and acetone (to a far lesser extent); β-hydroxybutyrate (BHB) is not detected. The ketones react with sodium nitroprusside which forms a purple color change. The color change is quite subtle, leading to many false positive reactions; this is due to the strong color of animal urine. The Cornell University Clinical Pathology Laboratory performed internal studies which have shown that most trace reactions (5 mg/dL) are false positive results. We also found that some small (15 mg/dL) to moderate (50 mg/dL) results may also be false positive reactions. Measurement of urinary β-hydroxybutyrate on our chemistry analyzer can be done to confirm any positive ketone result (this urinary test result is best interpreted concurrently with serum BHB concentrations). We also are confirming reactions that are small or larger (15 mg/dL or larger) with the Acetest®. Note that ketonuria will precede a ketonemia. By the time ketones have increased sufficiently in blood to cause a high anion gap titration acidosis, a ketonuria should be evident.
The table below illustrates the reportable ketones results from the CLINITEK Advantus Urine Chemistry Analyzer used at Cornell University.
Ketones
(mg/dL) |
Negative |
Trace |
15 (Small) |
40 (Moderate) |
≥80 (Large) |
Ketonuria indicates deranged energy metabolism, where fat is used instead of glucose for energy production. This usually occurs in states of negative energy balance, where energy intake does not meet demands. Fat metabolism can result in production of ketone bodies in amounts greater than can be metabolized by peripheral tissue; filtration into urine in excess of tubular reabsorption then results in ketonuria. Some specific causes include:
- Unregulated diabetes mellitus in dogs and cats
- Pregnancy toxemia in sheep and camelids
- Bovine ketosis (type I)
- Starvation or malnutrition, especially in immature animals
Acetest®
This tablet test is used to verify positive reactions from the dipstick, as the color change on the dipstick can be quite subtle. The Acetest® contains lactulose which enhances the color change and the result is considered to be more accurate than the dipstick, in which false positives can occur due to the color of the urine. The Acetest® is also useful for semi-quantitatively measuring ketones in other fluids, such as plasma, serum and milk.
Bilirubin
The bilirubin pad on the multireagent dipstick detects bilirubin using a specific diazotization reaction and is sensitive to 0.2-0.4 mg/dL of conjugated bilirubin. However, the color change indicating a positive reaction is a rather subtle transition among shades of beige, and sometimes is obscured by color inherent in the urine itself (e.g., dark yellow urine). In such instances, confirmation of a suspected positive reaction used to be done using a tablet version of the test, the Ictotest method, which yielded a purple color reaction which was easier to read. When there was a discrepancy between the dipstick bilirubin result and the Ictotest (e.g. small reaction on the dipstick and negative on the Ictotest®), the Ictotest® is considered the accurate result (i.e. a negative result in this example). In our experience, false positive reactions for bilirubin with the dipstick are uncommon (results of the dipstick and tablet versions of the test usually matched).
The table below illustrates the reportable bilirubin results from the CLINITEK Advantus Urine Chemistry Analyzer used at Cornell University.
Bilirubin |
Negative |
Small |
Moderate |
Large |
Positive reactions
- Physiologic: Dogs have a low renal threshold for bilirubin, therefore highly concentrated urine samples from this species may have a trace to 1+ reaction. Male dogs can also conjugate bilirubin in their renal tubules (after uptake of unconjugated bilirubin from blood) so male dogs are more likely to have a bilirubinuria than female dogs. This may be clinically insignificant. The same is true of ferrets, in that healthy ferrets can have small amounts of bilirubin in their urine (Orcutt 2003). In all other species, bilirubinuria indicates cholestasis.
- Cholestatic hepatobiliary disease: Detection of bilirubin in urine is generally an abnormal finding. Bilirubinuria generally results when conjugated bilirubin levels in blood are increased as a result of cholestatic hepatobiliary disease. Bilirubinuria (in excess for the USG) is expected to precede bilirubinemia and may maintain total (and direct) bilirubin concentrations within reference intervals in early cholestasis.
- Intravascular hemolytic anemia: In some cases of intravascular hemolytic anemia, bilirubinuria may be secondary to the hemolytic anemia without any evidence of cholestasis (this is very rare). The renal tubular epithelium is capable of absorbing free hemoglobin from the glomerular filtrate and converting it to conjugated bilirubin, which is then excreted in the urine. This will only occur with intravascular hemolysis, when free hemoglobin is filtered by the glomerulus (note the conjugated bilirubin is unlikely to be transported back into blood in this situation). However, most of the bilirubin in this scenario is due to concurrent cholestasis in affected animals.
Note that in chronic cholestasis, direct or conjugated bilirubin can bind to plasma proteins, such as albumin. Once protein bound, the direct bilirubin will no longer be filtered in the urine. Protein-bound conjugated bilirubin is called delta bilirubin and is one explanation for an increase in total and direct bilirubin without bilirubinuria, particularly in dogs, where the renal threshold for conjugated bilirubin is low (this is not considered a false negative reaction as the conjugate bilirubin is not being filtered).
False negative reactions
- Bilirubin crystals: In some samples, we have observed bilirubin crystals in the urine (indicating bilirubinuria), however the dipstick reaction for bilirubin is negative. The reason for this is unknown, however we speculate that crystals may not solubilize sufficiently to react with the dipstick in these cases, resulting in a false negative reaction.
- Aged urine samples: Conjugated bilirubin hydrolyzes to unconjugated bilirubin if left at room temperature.
- Exposure to UV light: UV light converts bilirubin to biliverdin, resulting in false negative reactions.
- Ascorbic acid: High concentrations of vitamin C inhibit the reaction.
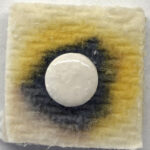
Ictotest®
The Ictotest® uses the same chemical reaction as the bilirubin pad on the multi-reagent dipstick, but is presented in a tablet format. The main advantage is that observation of the true color reaction is less affected by the inherent color of the urine itself. It is, therefore, useful in confirming or refuting apparently positive reactions on the dipstick in cases where the urine sample is deeply colored. It is also more sensitive to bilirubin than the dipstick (detects 0.1 mg/dL of bilirubin). At Cornell University, we historically used this test to confirm positive bilirubin reactions on the dipstick, this result being a more accurate assessment of urinary bilirubin. In reality, we hardly ever saw false positive bilirubin reactions with the dipstick (positive with the dipstick but negative on the Ictotest®). We did, however, occasionally see false negative reactions on the dipstick (negative on the dipstick, positive on the Ictotest®). These false negative reactions primarily occurred when bilirubin was only present in crystal form (i.e. bilirubin crystals were seen on urine sediment examination). The Ictotest® is no longer available. Internal studies at Cornell University have shown that measurement of bilirubin on our chemistry analyzer provides accurate results for bilirubin concentration in urine.
Heme
“Blood” on the dipstick represents the reaction observed when the “peroxidase-like” activity inherent in molecules of heme (iron within a porphyrin ring) reacts with a peroxidase substrate in the pad. Heme is found within hemoglobin (free in the urine or within erythrocytes) or myoglobin.
A positive reaction for heme on a dipstick occurs in the following situations:
- Hemorrhage (hematuria): This is the most common cause of a positive reaction. The reaction for heme on the dipstick is very sensitive and will detect heme associated with as few as 10 RBC/uL.
- Intravascular hemolysis (hemoglobinuria)
- Skeletal muscle injury (myoglobinuria)
- False positive reaction due to non-heme peroxidases: Bacterial peroxidases, chemicals with peroxidase activity (e.g. bleach).
We use clinical signs, other laboratory data (hemogram and clinical chemistry) and various attributes of urine (urine color, findings in urine sediment, taking into account method of urine collection) to differentiate between the three potential pathologic causes of positive heme reactions on the dipstick (hematuria, hemoglobinuria, myoglobinuria) and have given guidelines below. Usually, this can be accomplished readily, however there are occasions where we cannot readily explain a heme reaction (no RBC in the sediment, no intravascular hemolysis, no evidence of muscle injury). In such cases, it is possible that the heme reaction is a false positive due to peroxidase activity from bacteria or other chemical compounds or because the RBC that were in the urine have lysed. In rare cases, there may be more than one cause for a positive reaction for heme on the dipstick, e.g. hematuria and hemoglobinuria can occur concurrently, e.g. a dog with intravascular hemolysis and hemorrhage secondary to cystitis. In the latter cases, the heme reaction could be due to RBC from hematuria and hemoglobin from intravascular hemolysis. Similarly, an animal can have red urine with no RBC in the sediment and the heme reaction could be due to both myoglobin and hemoglobin, e.g. a horse with intravascular hemolysis secondary to oxidant injury from red maple leaf toxicity and with severe muscle injury. It becomes academic to try and determine if the red urine is due to hemoglobin or myoglobin. Both will cause renal injury from direct nephrotoxicity and hypoxic damage due to scavenging of nitric oxide (an important renal vasodilator). The guidelines given below do not permit direct distinction between hemoglobinuria and myoglobinuria (they are indirect methods of assessment). This can only be done with additional tests, e.g. electrophoresis, but is rarely required.
Note that the test is called “Heme” on our urinalysis results, which reflects what the test is detecting versus “blood”. The table below illustrates the reportable heme results from the CLINITEK Advantus Urine Chemistry Analyzer used at Cornell University.
Heme |
Negative |
Trace |
Small |
Moderate |
Large |
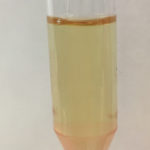
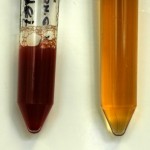
Distinguishing between true causes of a positive heme reaction on a dipstick
There are three main causes of a positive heme reaction on a dipstick: Hematuria, hemoglobinuria and myoglobinuria. Below are ways to differentiate between these causes, including a table summary.
- Hematuria: The dipstick is actually detecting hemoglobin within RBC, that lyse when they contact with the reagent pad. In some cases, a speckled pattern results if only some RBC are lysed when they contact the pad (called “non-hemolyzed” on the dipstick). Hematuria can be macroscopic (i.e. the urine is visibly red) or only microscopic (the urine is not visibly red, but RBC are seen in the sediment). With substantial hematuria, the urine pellet will be red (RBC precipitate) after centrifugation of the urine to examine the sediment. The urine supernatant is then usually clear (all the RBC are in the pellet) but could be red (if the RBC have lysed or there is concurrent hemoglobinuria or myoglobinuria). Again, this is the most common reason for a positive reaction for heme on a dipstick (which is why the term “blood” is likely used on some dipsticks to reflect a positive reaction for heme).
- Expected urine sediment findings: Intact RBC. One can attribute a positive heme reaction to hematuria if intact RBC are seen in the sediment (regardless of whether the urine is visibly red). If we see a positive reaction for heme on the dipstick and RBC in the sediment, we usually attribute the heme reaction to hematuria versus looking for another cause (hemoglobin from intravascular hemolysis or myoglobin from muscle injury). However, there are several occasions when RBC are not seen in the urine sediment despite the presence of hematuria. This could be due to lysis of RBC. With storage of urine, RBC that have entered the urine will lyse and a positive reaction for heme may occur in the absence of visible RBC in the sediment. This lysis is accelerated in urine with low specific gravity, i.e. <1.010 units, or high pH, usually ≥8.0 units. Note, that even though RBC are lysing in the urine and releasing their hemoglobin which is reacting with the dipstick, we do not use the term hemoglobinuria for these findings. Rather the term hemoglobinuria is used to indicate the hemoglobin is in urine from spill-over from free hemoglobin in plasma (from intravascular lysis of RBC in blood and not from lysis of RBC in urine).
- Expected hemogram and chemistry findings: No anemia (some animals may be anemic from other causes) and no hemoglobinemia; no evidence of severe muscle injury clinically or on the chemistry panel (muscle enzyme activities, e.g. CK, are not substantially increased). A positive reaction for heme on a dipstick in an anemic animal with no RBC in the urine sediment should not be used as evidence that the animal has hemoglobinuria and thus anemia due to intravascular hemolysis. The anemia in such animals can be due to multiple other causes, including suppression from inflammatory disease.
- Causes of hematuria: Hematuria results from bleeding into the urine and can occur at any level of the urinary or reproductive tract, i.e. indicates urogenital bleeding. However genital bleeding should only impact urine results in samples collected by free catch (voided) or catheter. Hematuria in urine samples collected by cystocentesis should reflect hemorrhage into the urinary tract (kidney, ureters, bladder).
- Iatrogenic hemorrhage occurs secondary to the collection procedure, i.e. cystocentesis or catheterization (only if traumatic or the urethra is already irritated or fragile with the latter). We frequently see microscopic hematuria as a consequence of cystocentesis if small vessels are damaged during the procedure. The hematuria is called microscopic because the urine is not discolored (cannot tell there is blood in there by looking at it grossly) but there are variable numbers of RBCs (>5 RBC/HPF) in the sediment; in some cases, many RBCs (>100 RBC/HPF) may be seen. It is difficult to distinguish iatrogenic from true hemorrhage and the possibility of iatrogenic hemorrhage should always be considered if urine is collected by cystocentesis. It is less likely in catheterized and unlikely in voided urine.
- Pathologic hemorrhage occurs due to genitourinary disease, e.g. inflammation, irritation from uroliths, neoplasia (transitional cell carcinoma in prostatic urethra or bladder), and hemostatic disorders. As indicated above, hemorrhage from the reproductive tract will not usually result in hematuria in a urine sample collected by cystocentesis.
- Hemoglobinuria: In this case, the hemoglobin is due to “spill-over” from excess free hemoglobin in plasma (i.e. hemoglobin concentrations in plasma high enough to saturate haptoglobin, so the hemoglobin overcomes the renal threshold and spills into the urine). This only occurs with intravascular hemolysis and associated causes of anemia due to intravascular hemolysis. The urine (before and after centrifugation) is usually grossly red (it may be light red) and no RBC are seen in the pellet (pellet is not red) when the urine is centrifuged for sediment examination (unless there is concurrent hematuria). Note that hemoglobin will oxidize in urine and become methemoglobin, so urine containing methemoglobin will be more brown than red.
- Expected urine sediment findings: No RBC.
- Expected hemogram and chemistry findings: Anemia (decreased HCT) with evidence of hemoglobinemia (red plasma). A cause of intravascular hemolysis may be evident, e.g. oxidant injury (eccentrocytes, Heinz bodies), specific infectious agents (e.g. Babesia). Extravascular hemolysis will not result in hemoglobinuria (thus hemoglobinemia and hemoglobinuria help distinguish between intra- and extravascular hemolytic anemias). There should be no clinical or laboratory evidence of severe muscle injury (i.e. CK should not be very high). As indicated above, a positive reaction for heme on a dipstick in an anemic animal with no RBC in the urine sediment does not indicate by itself that the animal has hemoglobinuria and intravascular hemolysis. There must be additional evidence to support this (see above).
- Causes of “true” hemoglobinuria: Any cause of intravascular hemolytic anemia
- Beware of “pseudo-hemoglobinuria”: This occurs when there is a heme reaction on the dipstick (usually large), which cannot be explained by myoglobin (no evidence of muscle injury), hematuria (no RBCs in the sediment) or intravascular hemolytic anemia. We do see this commonly in urine and it is attributed to the extreme sensitivity of the dipstick to RBCs which have likely lysed in the urine (e.g. with storage, high pH or dilute urine).
- Myoglobinuria: In this case, the positive reaction on the dipstick is due to “spill-over” from excess myoglobin in plasma. Myoglobin is not normally seen in plasma and is only released in high quantities with severe skeletal muscle injury (it is a large protein after all). The urine may be orange, red to brown (before centrifugation and supernatant after centrifugation) and no RBC will be seen in the pellet after centrifugation (unless the animal has concurrent hematuria). Note a brown urine color does not indicate myoglobin is in the urine. Hemoglobin rapidly oxidizes in urine and will be converted to methemoglobin, which is also brown. In humans, myoglobin is rapidly cleared (within several hours) from blood via renal clearance primarily (its molecular weight is well below the renal threshold at 16 kD) with some also being metabolized to bilirubin. Myoglobin appears in urine when concentrations in plasma are >1.5 mg/dL. A change in urine color will occur if the myoglobin is >100 mg/dL (Huerta-Alardin et al 2004).
- Expected urine sediment findings: No RBCs.
- Expected hemogram and chemistry findings: No anemia (unless animal has another cause of anemia) or hemoglobinemia (unless concurrent intravascular hemolysis). There should be clinical (stiffness, pain) and laboratory evidence of severe muscle injury (i.e. very high CK activity, e.g. >50,000 U/L, AST and LDH may be concurrently increased unless injury very acute).
- Causes: Severe muscle injury, usually affecting skeletal muscle (which has the largest muscle mass), e.g. exertional rhabdomyolysis in horses (“tying up”), inherited myopathies in dogs. Injury affecting smooth muscle (e.g. gastrointestinal tract) or cardiac muscle is unlikely to result in sufficient myoglobin release to cause myoglobinuria.
Table summary heme reactions
Explanation | Urine color | Supernatant color | Pellet color | RBC in sediment | Anemia | Hemoglobinemia* | Evidence of muscle injury (very high CK)** |
Hematuria (intact RBC) | May be red | Not red | May be red | Yes | No*** | No | No |
Hematuria (all RBC lysed in urine) | May be red | May be red | Not red | No | No*** | No | No |
Hematuria (intact plus lysed RBC) | May be red | May be red | May be red | Yes | No*** | No | No |
Hemoglobinuria from intravascular hemolytic anemia | Likely red | Likely red | Not red | No | Yes | Yes | No |
Myoglobinuria | Likely (usually red/brown) | Likely (usually red/brown | Not red | No | No*** | No | Yes |
Urine = urine before centrifugation; supernatant and pellet = after centrifugation of urine to create a urine sediment (pellet), may be = depends on extent or degree of hematuria.* Note, this refers to hemoglobinemia due to intravascular hemolysis. Artifactual in vitro hemolysis (RBC lysing in the collection tube or lysing during collection, but not lysing in in the animal) will also result in red plasma, however in vitro hemolysis will not cause hemoglobinuria (see table for differentiating between in vivo and in vitro hemolysis under mechanisms of anemia)!** Mild muscle injury (as evidenced by a mildly increased CK activity) is not expected to result in substantial myoglobinuria.***Animals may be anemic for other reasons (which may depend on the primary underlying disease). Also substantial or chronic blood loss from the genitourinary tract can result in a blood loss anemia (an iron deficiency anemia can also ensue from chronic blood loss once iron stores are depleted). |
Uric acid
Uric acid is formed from the catabolism of the nucleic acids, adenine and guanine. Both of these nucleic acids are converted to xanthine, guanine directly and adenine after being converted to hypoxanthine first. Xanthine is converted to uric acid via the action of xanthine oxidase. Uric acid is usually converted by hepatic uricase to allantoin, which is excreted in the urine of most dog breeds, except the Dalmation. The Dalmation has a defect in a putative uric acid transporter, SLC2A9 (Bannasch et al 2008), which results in decreased uric acid uptake into hepatocytes and decreased conversion to allantoin. Therefore, this breed excretes uric acid, and not allantoin, in the urine. Similar defects have been reported in Black Russian Terriers and English Bulldogs (Karmi et al 2010). The supersaturation of uric acid, which can take the form of ammonium biurate crystals) in the urine of these dogs predisposes these breeds to urate urolithiasis (Barges et al 1994, Houston et al 2004). Measurement of uric acid in urine is used to detect excessive urate excretion in breeds, such as Dalmations, and to monitor response to therapy with allopurinol. The best method for measuring urinary urate excretion is from a 24-hour urine collection. Uric acid precipitates rapidly when urine is cooled, so the sample must be resuspended and a well-mixed aliquot provided to the laboratory, to prevent falsely decreased values due to precipitation. The determination of a uric acid/creatinine ratio does not appear to be a reliable test for quantifying urinary uric acid excretion, as the values are influenced by diet.
Urobilinogen
Urobilinogen is produced in the intestine from bacterial action on excreted bililrubin from bile. Some is degraded to stercobilinogen, which is excreted in feces, but the rest is reabsorbed taken up by the liver, with excess being excreted in the urine. Decreased urobilinogen (urobilin) is seen with cholestasis (does not enter the intestine to be produced) or dysbiosis (decreased produced in intestine), whereas high values can be seen in liver disease (decreased uptake or cycling) and hemolytic anemia (increased production and excretion of conjugated bilirubin into the intestine, if there is no concurrent cholestasis). In general, this test is not considered diagnostically useful in animals.
References
- Miki et al 2011: J Vet Med Sci. Based on a significant increase in urine glucose to creatinine ratios in healthy beagles given intravenous glucose (although values were still quite low).
- Eggleton and Schuster 1954: J Physiology. Based on the finding that most of the filtered glucose was resorbed (with <10 mg remaining) in urine with serum glucose < 270 mg/dL after intravenous administration of glucose. At higher glucose concentrations (>450 mg/dL), less than 50% of the glucose was resorbed in the kidney in some cats.