Interpretation
Oxidant injury: Methemoglobinemia and Heinz body formation; presumptive acetaminophen toxicosis
Explanation
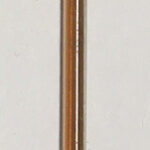
The submitted blood was brown, with a brown supernatant on centrifugation (see image to right), supporting methemoglobinemia with oxidation of iron (to Fe3+) within the heme ring of hemoglobin. A smear made from the submitted sample showed storage-associated changes in white blood cells, such as swelling of the nuclear chromatin of neutrophils and cell smudging, and red blood cells (echinocytosis, lysis with ghost cells). Platelets were clumped but deemed adequate (Figure 4), verifying the automated platelet count. A manual differential count consisted of 85% neutrophils (reference interval [RI], 27-82%), 11% lymphocytes (RI, 9-56%), 1% monocytes (RI, 0-6%) and 3% eosinophils (RI, 1-15%) with no basophils (RI, 0-2%), indicating that the analyzer had over-estimated neutrophil and basophil percentages and underestimated lymphocyte and eosinophil percentages (Figures 1-2). Regardless, both automated and manual results indicated a neutrophilia (absolute neutrophil count of 12.8 x 103/μL, RI, 2.3-11.6 x 103/μL), either due to mild inflammation or stress (endogenous corticosteroid release). Blood smear examination revealed many small Heinz bodies, which were difficult to see on most of the RBCs, consisting mostly of small pale-staining circular regions (Figure 5, Question 1). The Heinz bodies were also seen extracellularly (from ruptured cells) and were more obvious in lysing or ghost RBCs or at the feathered edge of the smear, where “free” Heinz bodies are found in higher numbers (Figures 4-6). There were also a few polychromatophilic RBCs and keratocytes. Low numbers of both of the latter cell types can be seen in the blood of healthy cats.
Heinz bodies are easier to visualize using a new methylene blue-stained smear (Question 2). In this case, the dye was incubated with an equal volume of blood to make a permanent smear and showed small blue Heinz bodies on 80-90% of the RBCs and also free in the background and in lysing RBCs (Figure 7). The new methylene blue-stained smear also highlighted punctate reticulocytes (Figure 7). A manual reticulocyte count was done on the new methylene blue-stained smears and yielded <1% aggregate reticulocytes and 3% punctate reticulocytes (RI, 0-7.4%1). The gross and microscopic features of the blood indicated oxidant injury to RBCs, with precipitation of hemoglobin forming Heinz bodies and oxidation of iron within the heme ring causing methemoglobinemia. Considering the age of the cat, the primary differential diagnosis was acetaminophen toxicosis (Question 3).
|
The biochemical panel revealed a moderate to marked hyperglycemia compatible with stress (endogenous glucocorticoids or epinephrine). The mild hypokalemia could be secondary to losses from vomiting combined with decreased intake from inappetence. Intracellular translocation (if the cat had alkalemia from a primary respiratory alkalosis caused by hypoxemia, considering the panting) and urinary losses (increased aldosterone if there was a stimulus for the renin-angiotensin-aldosterone system) of potassium cannot be ruled out.
Additional tests
The fraction of hemoglobin that was methemoglobin (fmetHb) was determined by pulse oximetry on a Radiometer blood gas analyzer (ABL Flex 90). Triplicate measurements on the sample yielded a fmetHb of 43.7% versus 3.1% in a control sample from another cat. These results confirmed methemoglobinemia. A high concentration of acetaminophen was detected with high performance liquid chromatography in serum submitted from the cat, confirming acetaminophen toxicosis. In theory, this testing is not routinely done, with the diagnosis being made based on history and associated clinical signs of cyanosis and brown mucosa and blood (Question 4).
Discussion
Acetaminophen (N-acetyl-p-aminophenol) is a rapidly acting short lasting anti-pyretic and painkiller that is generally safe and effective in human beings. The drug is rapidly absorbed after oral ingestion, with 11% being bound to plasma proteins in dogs. It is taken up and metabolized primarily in the liver to non-toxic glucuronide and sulfate conjugates. Both unbound drug and conjugated metabolites are excreted via the kidney, with glucuronide- and sulfate- conjugates dominating in urine of all species (humans, rats, mice, hamsters, guinea pigs), except for the cat, which excretes 89% of acetaminophen as unknown metabolites, with approximately 3% unmetabolized unbound drug.2 Some of the conjugated metabolites are also excreted into the biliary system. Due to conjugation, only a small amount of the parent drug is oxidized to a hepatotoxic metabolite by the cytochrome P-450 complex. This toxic metabolite is primarily thought to be N-acetyl-p-benzo-quinoneimine (NAPQI).3 The NAPQI is detoxified by conjugation to glutathione (GSH) by glutathione S-transferase and is excreted in the urine as a mercapturic acid-conjugate. However, when GSH is depleted, it covalently binds to lipid membranes, particularly in mitochondria, with ensuing production of oxidants (peroxynitrite) and subsequent mitochondrial injury-mediated necrosis of centrilobular hepatocytes.2-3 Liver toxicity is correlated to glutathione (GSH) depletion and occurs when GSH is decreased to 20-30%. Depletion of GSH occurs to varying degrees in different species (e.g. mice are more sensitive to GSH reductions than rats) and even within individuals of the same species to the same dose of drug,2-4 explaining the wide range of toxic doses seen in individual animals (10-400 mg/kg in cats5). Although NAPQI is considered the main hepatotoxic metabolite, this compound does not appear to be the cause of methemoglobinemia. Rather the p-aminophenol portion of the drug may be responsible for methemoglobinemia in dogs and cats. In vitro incubation studies showed increased susceptibility of canine and feline RBCs to the oxidizing effects of p-aminophenol, but not the parent compound or NAPQI.6 How p-aminophenol is produced from the parent drug is unclear, but an alternative metabolic pathway involving deacetylation is thought to be involved.7 The increased sensitivity to p-aminophenol in dogs and cats versus rodents was attributed to a relative deficiency of the drug metabolizing enzyme, arylamine N-acetyltransferase.8,9 This enzyme helps detoxify drugs, by transferring an acetyl group from acetyl-CoA to the drug.
In humans, toxicity is primarily related to the gastrointestinal tract and liver, with rare cases of methemoglobinemia and neurologic symptoms (e.g. coma, convulsions). Acute signs of toxicity (within 5-24 hours) are gastrointestinal in origin, such as nausea and vomiting. In some individuals, liver toxicity becomes evident within 2-4 days after ingestion. Some patients, typically those with liver toxicity, also develop acute renal tubular necrosis, which is attributed to the p-aminophenol portion of the drug.2 In one toxicity study in dogs and cats, the same animals were given 100, 200 and 500 mg/kg and 20, 60 and 120 mg/kg of acetaminophen with a 2 to 3 week washout between escalating doses, respectively. Methemoglobinemia (>5%) with pigmenturia was seen in dogs dosed with 200-500 mg/kg and one animal died within 24 hours of being given the highest dose. The higher doses also caused vomiting, depression and facial, paw and foreleg edema. Similarly, methemoglobinemia (and pigmenturia) was seen in cats given the intermediate dose of 60 mg/kg, but all cats given the highest dose showed clinical signs of toxicity, including depression, anorexia, vomiting or salivation, facial edema and methemoglobinemia, within 8 hours. The edema in some dogs and cats lasted for several days. High drug dosages yielded methemoglobin concentrations similar to that seen in the cat of this report (46% within 12 hours in cats) and GSH RBC concentrations decreased by 77% and 82% from starting concentrations in dogs and cats, respectively, with the largest decrease being seen in the dog that died (1% of starting concentration). Drug clearance was delayed in cats with higher doses, suggesting decreased metabolism and, of the tested metabolites, sulfate-conjugated forms dominated in urine with very few glucuronide-conjugates (5% of the concentration in the urine of dogs with similar doses). Cats also demonstrated saturation of sulfate-based detoxification with decreasing amounts of urine sulfated conjugates with increasing doses. There was evidence of liver and renal toxicity in dogs at the highest dosage, with increased ALT and ALP activities on biochemical analysis and centrilobular necrosis, cholestasis, protein casts and nephrosis identified on histologic evaluation of tissues obtained post-mortem.4 Hepatocytes were vacuolated, with no evidence of necrosis, in the liver of the cats, but renal damage was not evident histologically at post-mortem examination. Two of 6 cats had evidence of lung edema,4 which may contribute to the respiratory distress seen in many cats with accidental toxicosis.5 Similar findings were reported in another experimental study, where cats were given 150 mg/kg acetaminophen orally. Cats developed acute cyanosis and tachypnea (within 2-6 hours) with later depression and anorexia (24-72 hours). Activities of ALT were high at 4 hours and peaked at 24 hours, but were still increased at 72 hours, indicating hepatotoxicity. Methemoglobin concentrations increased acutely at 4 hours, followed by a rapid decline at 24 hours, corresponded to decreased liver and RBC GSH concentrations.10
In 17 cats with naturally occurring toxicosis, toxicity was evident at doses as low as 10 mg/kg, supporting marked individual variability in susceptibility to toxicity. The increased susceptibility of cats to acetaminophen toxicosis is attributed to their inherent reduced activity of the uridine 5’-diphospho (UDP)-glucuronyltransferase enzyme in hepatic microsomes11-12 and reduced sulfate-based conjugation,4 resulting in increased production of toxic metabolites. Deficiency of arylamine N-acetyltransferase, which is suspected to detoxify p-aminophenol, likely explains the methemoglobinemia.6,8 The UDP glucuronyltransferase isoform (1A6) that is responsible for conjugating acetaminophen to glucuronide is not expressed in cats due to silencing gene mutations (however, the isoform that conjugates bilirubin, UGT1A1, is still expressed).12 Species-related differences in the drug transporter gene, the G2 subfamily of the ATP-binding cassette subfamily (ABCG2), has also been speculated to play a role. This gene is responsible for biliary excretion of sulfated-conjugates of acetaminophen and has reduced functionality in cats due to amino acid polymorphisms at conserved sites. Decreased biliary secretion may increase the amount of acetaminophen that is metabolized to the toxic form by P-450 enzymes.13 Increased concentrations of the mercapturic acid-conjugate are seen in the urine of cats versus dogs, supporting increased production of the toxic metabolites in cats.4
In the case series of 17 cats with natural toxicosis, cats typically were presented to the veterinarian within 24 hours of drug ingestion and the most common clinical signs were marked depression and respiratory distress with about 1/3 of cats displaying anorexia, vomiting, facial/paw edema and salivation. Neurologic symptoms, such as coma and ataxia, were uncommon. Many cats were tachypneic, with pale or brown mucosa and hypothermia. A mild to severe anemia (9-29% hematocrit) was detected in 76% of the cats on clinical pathologic testing and, as in this case, 2 cats had many Heinz bodies. Around 1/3 of cats had evidence of liver injury (increased ALT activity, low cholesterol concentration), with 2 cats having hyperbilirubinemia. Several cats had gross or dipstick-based pigmenturia (urinalysis was only done in 2 cats).5 Even though the cat of this report was not anemic, ghost RBCs and “free” methemoglobin was present in plasma, suggesting that there a degree of intravascular hemolysis occurring, albeit insufficient to result in an anemia. This speculation cannot be confirmed because the plasma appearance at presentation to the emergency clinic was not ascertained and urine was not obtained from the cat. A compensated hemolytic anemia was considered unlikely because neither aggregate nor punctate reticulocyte percentages were increased. Ghost RBCs and increased free methemoglobin in plasma could also have been an artifact of delayed sample storage with increased instability or fragility of the RBCs due to oxidant injury. Heinz bodies in this cat were small and difficult to visualize, being more readily identified in ghost RBCs or as “free” structures at the feathered edge, where they tended to concentrate. These smaller Heinz bodies mimic those seen in anemic or non-anemic cats and are so-called “endogenous” Heinz bodies. Higher numbers of these Heinz bodies occur in cats with diseases or conditions associated with oxidant production, including diabetes mellitus, hyperthyroidism and lymphoma (gastrointestinal T cell, in particular),14-15 or ingestion of foods containing onion powder or propylene glycol.16-17 In one study, cats with Heinz bodies had lower packed cell volumes than control cats without Heinz bodies,14 suggesting that extravascular hemolysis (reduced RBC lifespan) is occurring, however not all affected cats are anemic, as seen in this case. Cats with Heinz bodies also had slightly lower GSH concentrations.14 Interestingly, Heinz bodies were seen more frequently in older cats, 16 which could be due to the increased likelihood of associated diseases in such cats or decreased GSH concentrations with age, as seen in mice.2 Feline hemoglobin contains around 8 oxidant prone sulfhydryl groups,18 rendering it more susceptible to oxidant injury. Many Heinz bodies will also interfere with results of some hematologic tests, including hemoglobin (and hemoglobin-related indices, including MCH and MCHC) as measured by standard spectrophotometric methods with all analyzers, and the WBC count with the ADVIA series of hematology analyzers in some patients.19
Differential diagnoses for methemoglobinemia in cats include acetaminophen toxicosis, other oxidants such as benzocaine,20 and an inherited or congenital deficiency in cytochrome b5 methemoglobin reductase, the enzyme responsible for reducing methemoglobin.21 The latter should be suspected in young cats presenting with cyanosis due to methemoglobinemia. In some cases, the oxidant is not identified.22 Methemoglobinemia is usually detected clinically by muddy brown mucosa and brown blood, which can be highlighted by the “blood spot” test, i.e. placing a drop of blood on a white piece of paper.22 It can be confirmed by oximetry (ideally co-oximetry) measurements as done in this case, which yielded the fraction of hemoglobin in the methemoglobin form. Of note, pulse oximetry measurements provide inaccurate (overestimated) results for oxygen saturation in the presence of methemoglobin (because oximetry uses two wavelengths to measure oxygenated hemoglobin [940-990 nm] and deoxyhemoglobin [660 nm], which includes methemoglobin [630 nm]), whereas pulse co-oximetry measurements, which uses multiple wavelengths, are more accurate in the presence of methemoglobin.23 Methemoglobin concentrations of >15% will result in clinical cyanosis.24 In this case, the high methemoglobin concentration, albeit measured in blood stored for 5 days, explained the clinical signs in this cat. Methemoglobin concentrations may decrease with storage (being more stable in hemolysates)25 and it is possible that the in vivo concentration was even higher than that obtained by our analyzer.
The treatment of cats with acetaminophen relies upon administration of N-acetylcysteine,5,26 which binds the toxic metabolites and is a glutathione precursor (Question 5). The protocol is an initial dose of 140 mg/kg IV, then 70 mg/kg intravenously every 6 hours for a minimum of 6-8 total doses.26,27 Emesis can be induced or activated charcoal administered with acute drug ingestion, however charcoal will inhibit absorption of orally administered N-acetylcysteine. N-acetylcysteine is quite unpalatable and needs to be mixed with a sweetener, like apple juice. If an oral glutathione donor is needed, Denaramin (a combination of S-adenosylmethionine [SAM-e] and silybin [an extract of milk thistle that is thought to help with liver toxicity] can be given orally, but there are no clinical or experimental studies of efficacy. Other anti-oxidants, such as vitamin C, are of unproven efficacy.26
Follow-up
In this case, N-acetylcysteine was administered intravenously at 140 mg/kg as a loading dose followed by repeated doses at 70 mg/kg. The cat recovered uneventfully and was discharged to the owner. Considering the degree of methemoglobinemia and delayed onset of therapy, this cat has certainly used up one of its nine lives.
References
- Harvey JW 2012. Veterinary Hematology: A Diagnostic Guide and Color Atlas. Elsevier Saunders. ISBN: 978-1-4377-0173-9, pp 91-93.
- Savides MC and Oehme FW. Acetaminophen and its Toxicity. J Applied Toxicol 1983; 3(2):96-111
- Jaeschke H, McGill MR, Williams CD, et al. Current Issues With Acetaminophen Hepatotoxicity–A Clinically Relevant Model to Test the Efficacy of Natural Products. Life Sci 2011; 88(17-18):737-745. DOI: 1016/j.lfs.2011.01.025
- Savides MC, Oehme FW, Nash SL, et al. The Toxicity and Biotransformation of Single Doses of Acetaminophen in Dogs and Cats. Toxicol Applied Pharmacol 1984; 74:26-34.
- Aronson LR and Drobatz K. Acetaminophen Toxicosis In 17 Cats. J Vet Emerg Crit Care 1996;6(2):65-69.
- McConkey SE, Grant DM and Cribb AE. The Role of Para-Aminophenol in Acetaminophen-Induced Methemoglobinemia in Dogs and Cats. J Vet Pharmacol Ther 2009;32(6):585-595. DOI: 1111/j.1365-2885.2009.01080.x
- Mazaleuskaya LL, Sangkuhl K, Thorn CF, et al. PharmGKB summary: Pathways of acetaminophen metabolism at the therapeutic versus toxic doses. Pharmacogenet Genomics 2015; 25(8):416-426.
- Trepanier LA, Cribb AE, Spielberg SE, et al. Deficiency of Cytosolic Arylamine N-acetylation in the Domestic Cat and Wild Felids Caused by the Presence of a Single NAT1-like Gene. Pharmacogenetics 1998; 8(2): 169-179. DOI: 1097/00008571-199804000-00009
- Trepanier LA, Ray K, Winand NJ, et al. Cytosolic Arylamine N-acetyltransferase (NAT) Deficiency in the Dog and Other Canids Due to an Absence of NAT Genes. Biochem Pharmacol 1997; 54(1):73-80. DOI: 1016/s0006-2952(97)00140-8
- Denzoin Vulcano, Confalonieri O, Franci R, et al. Efficacy of free glutathione and niosomal glutathione in the treatment of acetaminophen-induced hepatotoxicity in cats. Open Vet J 2013; 3(1):56-63.
- Court MH and Greenblatt DJ. Molecular Basis for Deficient Acetaminophen Glucuronidation in Cats. An Interspecies Comparison of Enzyme Kinetics in Liver Microsomes. Biochem Pharmacol 1997; 53(7):1041-1047. DOI: 1016/s0006-2952(97)00072-5
- Court MH and Greenblatt DJ. Molecular genetic basis for deficient acetaminophen glucuronidation by cats: UGT1A6 is a pseudogene, and evidence for reduced diversity of expressed hepatic UGT1A isoforms. Pharmacogenetics 2000; 10:355–369.
- Mealey KL. ABCG2 Transporter: Therapeutic and Physiologic Implications in Veterinary Species. J Vet Pharmacol Therap 2012; 35(2):105-112. DOI: 1111/j.1365-2885.2011.01313.x
- Christopher MM. Relation of Endogenous Heinz Bodies to Disease and Anemia in Cats: 120 Cases (1978-1987) J Am Vet Med Assoc 1989; 194(8):1089-95.
- Christopher MM, Broussard JD and Peterson ME. Heinz Body Formation Associated With Ketoacidosis in Diabetic Cats. J Vet Intern Med 1995; 9(1): 24-31. DOI: 1111/j.1939-1676.1995.tb03268.x
- Christopher MM, White JG and Eaton JW. Erythrocyte Pathology and Mechanisms of Heinz Body-Mediated Hemolysis in Cats. Vet Pathol 1990; 27(5):299-310. DOI: 1177/030098589002700501
- Robertson JE, Christopher MM and Rogers QR. Heinz Body Formation in Cats Fed Baby Food Containing Onion Powder. J Am Vet Med Assoc1998; 212(8):1260-1266.
- Taketa F, Smits MR, Lessard JL. Hemoglobin heterogeneity in cats. Biochem Biophys Res Commun 1968; 30(3):219-226. DOI: 1016/0006-291x(68)90438-5
- Dondi F, Vasylyeva K, Serafini F et al. Heinz body–related interference with leukocyte and erythrocyte variables obtained by an automated hematology analyzer in cats. J Vet Diagn Invest 2019; 31(5):704-713. DOI: org/10.1177/1040638719863088
- Wilkie DA and Kirby R. Methemoglobinemia Associated With Dermal Application of Benzocaine Cream in a Cat. J Vet Am Med Assoc 1988; 192(1):85-86.
- Jaffey JA, Reading NS, Giger U et al. Clinical, Metabolic, and Genetic Characterization of Hereditary Methemoglobinemia Caused by Cytochrome B 5Reductase Deficiency in Cats. J Vet Intern Med 2019; 33(6):2725-2731. DOI: 1111/jvim.15637
- Cavana P, Irato E, Miniscalo B et al. Severe Heinz Body Anemia and Methemoglobinemia in a Kitten With Chronic Diarrhea. Schweiz Arch Tierheilkd 2018; 160(4):235-239. DOI: 17236/sat00156.
- Barker SJ, Tremper KK and Hyatt J. Effects of Methemoglobinemia on Pulse Oximetry and Mixed Venous Oximetry. Anesthesiology 1989; 70(1):112-117. DOI: 1097/00000542-198901000-00021
- Wright RO, Lewander WJ, Woolf AD. Methemoglobinemia: etiology, pharmacology, and clinical management. Ann Emerg Med 1999; 34(5):646-656. DOI: 1016/s0196-0644(99)70167-8.
- Sato K, Tamaki K, Okajima H et al. Long-term Storage of Blood Samples as Whole Blood at Extremely Low Temperatures for Methemoglobin Determination. Forensic Sci Int 1988; 37(2):99-104. DOI: 1016/0379-0738(88)90098-9.
- Richardson JA. Management of Acetaminophen and Ibuprofen Toxicoses in Dogs and Cats. J Vet Emerg Crit Care 2000; 19(4):285-291.
- Plumb’s Veterinary Drugs. Accessed on-line.
Authored by: T. Stokol, S Dickers, E Frye